Developmental Stress and Correlated Cognitive Traits in Songbirds
Tara Farrell
University of Western Ontario
Buddhamas Kriengwatana
Leiden University
Scott A. MacDougall-Shackleton
University of Western Ontario
Reading Options:
Continue reading below, or:
Read/Download PDF | Add to Endnote
Abstract
Early-life environments have profound influence on shaping the adult phenotype. Specifically, stressful rearing environments can have long-term consequences on adult physiology, neural functioning, and cognitive ability. While there is extensive biomedical literature regarding developmental stress, recent research in songbirds highlights similar findings in domesticated and non-domesticated species, opening up the field to broader questions with an ecological and evolutionary focus. Here, we review the literature in songbirds that exemplifies how developmental stress can shape birdsong, a sexually selected cognitive trait, and other physiological and cognitive abilities. Furthermore, we review how various traits can be correlated in adulthood as a result of various systems developing in tandem under stressful conditions. In particular, birdsong may be indicative of other cognitive abilities, which we explore in depth with current research regarding spatial cognition. In addition, we discuss how various personality traits can also be influenced by the intensity and timing of developmental stress (prenatal versus postnatal). We conclude by highlighting important considerations for future research, such as how assessing cognitive abilities is often constrained by experimental focus and more weight should be given to outcomes of reproductive success and fitness.
Author Note: Tara Farrell and Scott A. MacDougall-Shackleton, Department of Psychology and Advanced Facility for Avian Research, University of Western Ontario, 1151 Richmond Street, London, Ontario, Canada N6A 5B8; Buddhamas Kriengwatana, Institute of Biology Leiden (IBL), Leiden University, Sylviusweg 72, 2333 BE, Leiden, The Netherlands.
Correspondence concerning this article should be addressed to Tara M. Farrell at tfarrel2@uwo.ca.
Keywords: developmental stress; birdsong; cognition; correlated traits; hippocampus; corticosterone; behavioral syndromes
Organisms exhibit integrated phenotypes, with various traits working in concert to produce a functioning whole. Traits typically co-vary among individuals, and much research has attempted to determine the underlying causes of correlations between different traits. One such cause is the influence of environmental factors on developmental trajectories, which can induce pleiotropic effects on the adult phenotype. That is, environmental conditions do not impact the development of a single trait in isolation, but will affect numerous traits simultaneously, thus potentially leading to developmentally correlated traits (Metcalfe & Monaghan, 2001; Spencer & MacDougall-Shackleton, 2011). In the case of cognition and behavior, any neural developmental processes that are sensitive to environmental factors at the same time points in development may become developmentally correlated, even if they are functionally unrelated (Figure 1).
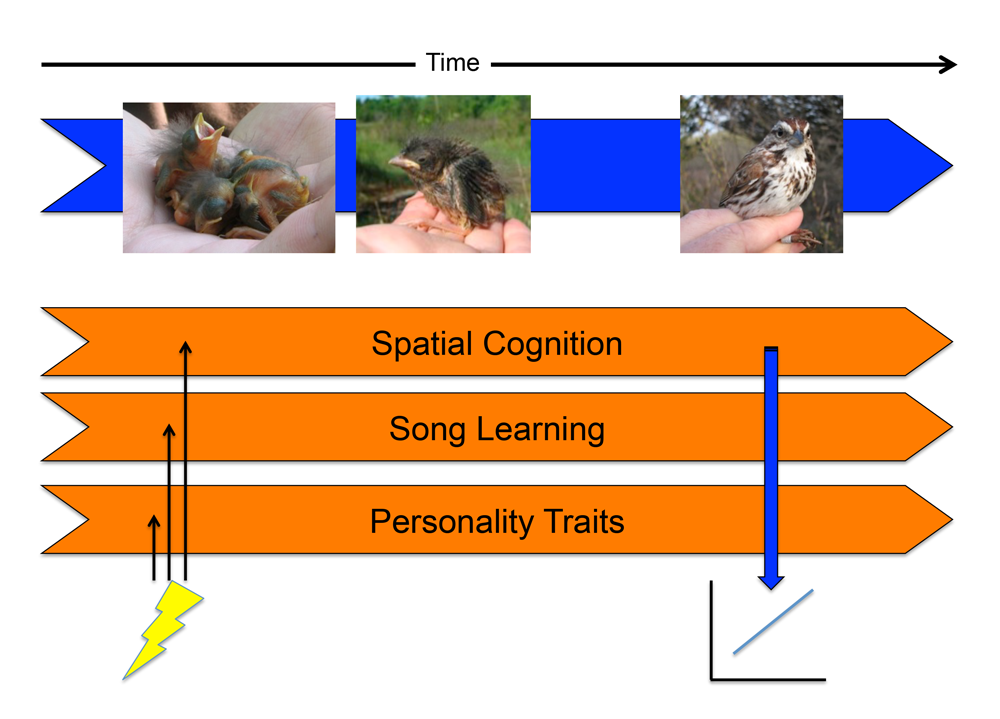
Figure 1. Developmental stress may induce correlations among traits in adulthood. Horizontal orange arrows indicate the developmental trajectories of neurocognitive systems. If a stressor affects the development of multiple neurocognitive traits early in development (indicated by lightning bolt), this may result in positive correlations between the traits in the adult animal (time point indicated by vertical blue arrow). In this way, traits that are functionally independent in adulthood (e.g., birdsong and spatial memory) may be correlated across individuals. Figure modified from Spencer & MacDougall-Shackleton (2011) with permission.
There has been growing interest in how stressful environmental factors can influence development. In one view, such developmental stressors can disrupt development and result in impaired performance. In another view, developmental stressors may program development to produce an adult better suited to a stressful environment (phenotypic programming; see Monaghan, 2008). If stressors impair development, then stressed individuals should have impairments in multiple traits. If stressors induce phenotypic programming, then stressed individuals should have multiple programmed traits. Regardless of these competing views, developmental stressors provide a potent mechanism by which multiple neural and cognitive traits may become developmentally correlated.
The aim of this review is to provide an overview of the literature regarding the effects of developmental stress on various cognitive-behavioral traits in songbirds. Cognition, defined for this review, refers to the processes that underlie the acquisition, processing, and storage of information, which an animal uses to interact within its environment (Shettleworth, 2009, p. 720). We explore how the developmental stress hypothesis—originally formulated to explain how developmental experiences can affect birdsong in particular—can be extended as a framework to help researchers understand how cognitive and behavioral traits in general may become correlated through developmental stressors. Consequently, this review will synthesize findings in songbirds regarding the developmental stress hypothesis, the implications that this hypothesis has for females, the reliability of song as a proxy of other cognitive abilities, and the effects of developmental stress on spatial cognition and personalities/behavioral syndromes—topics addressed by recent reviews of the developmental stress hypothesis that warrant a more thorough discussion (Buchanan, Grindstaff, & Pravosudov, 2013; MacDougall-Shackleton & Spencer, 2012; Spencer & MacDougall-Shackleton, 2011).
There are two primary reasons for focusing this review on songbirds despite the large and growing biomedical literature on the effects of early stressors on neural and cognitive development in domesticated species (e.g., Andersen & Teicher, 2008; Heim & Nemeroff, 2001; Heim, Shugart, Craighead, & Nemeroff, 2010; Welberg & Seckl, 2008). First, it is important to understand the role that stress plays in cognitive development in non-domesticated species because these studies provide valuable insights into the effects of developmental stress on ecologically relevant behaviors and sexual selection (e.g., MacDougall-Shackleton & Spencer, 2012; Buchanan et al., 2013). Second, birdsong is one of the most extensively studied areas of animal behavior and cognition, and depends critically on developmental experience. In addition, songbirds have been studied with respect to hippocampus-dependent spatial cognition, behavioral innovation, and behavioral syndromes, providing a rich and diverse animal model from which we can understand how stress affects a variety of cognitive functions.
Birdsong and the Developmental Stress Hypothesis
There has been a long-standing interest in the mechanisms of birdsong learning and development. Because birdsong is a sexually selected trait, there has also been extensive research on the types of information transmitted by birdsong and how song can act as an indicator signal to accurately provide information to the receiver regarding qualities of the singer. The current prevailing hypothesis to explain how song can be an honest signal of male quality is the developmental stress hypothesis (Nowicki, Hasselquist, Bensch, & Peters, 2000; Nowicki, Peters, & Podos, 1998). Because song learning and development of the neural structures underlying song occur over a protracted period of early life, they may be sensitive to a variety of stressors. Thus, a bird that sings a song of high quality is a bird that suffered relatively little stress during early life or was able to cope with such stress. Consequently, song may also provide predictive information about other traits that are sensitive to developmental stress (Nowicki et al., 1998). We will first review what is known about the effects of developmental stress on male song development and then discuss the effects of developmental stress on song learning and preference in the underrepresented female.
The developmental stress hypothesis has received substantial empirical support, as developmental stressors ranging from dietary manipulations, glucocorticoid administration, brood size manipulation, and immunological challenges have all been found to affect adult song and associated neurological structures (reveiwed in Buchanan et al., 2013; MacDougall-Shackleton & Spencer, 2012; Spencer & MacDougall-Shackleton, 2011). However, not all published experimental manipulations have found an effect of developmental stress on song learning. This is particularly evident in the multitude of zebra finch (Taeniopygia guttata) studies, where the effects of a variety of developmental stressors on song learning are inconsistent, with some studies reporting effects (Brumm, Zollinger, & Slater, 2009; de Kogel & Prijs, 1996; Holveck, Vieira de Castro, Lachlan, ten Cate, & Riebel, 2008; Spencer, Buchanan, Goldsmith, & Catchpole, 2003; Tschirren, Rutstein, Postma, Mariette, & Griffith, 2009; Zann & Cash, 2008), and others no effect (Gil, Naguib, Riebel, Rutstein, & Gahr, 2006; Kriengwatana et al., 2014).
The exact reasons for such discrepancies between studies, especially with zebra finches, remain unclear because the mechanisms by which developmental stress affects song have yet to be firmly established. Currently, developmental stressors are hypothesized to largely exert their effects by acting on the hypothalamic-pituitary-adrenal (HPA) axis. When stressors are perceived, the HPA axis mediates the physiological stress response by secreting glucocorticoids hormones (corticosterone [CORT] is the primary avian glucocorticoid). Thus, the way by which any type of developmental stressor affects song could be through activation of the HPA axis and the subsequent effects of CORT. For example, food restriction can alter baseline and stress-induced levels of CORT in birds (Kitaysky, Kitaiskaia, Wingfield, & Piatt, 2001; Pravosudov & Kitaysky, 2006) and elevated CORT due to food restriction can in turn adversely affect brain development (Welberg & Seckl, 2001). In support of this, some studies have found parallel effects of CORT and food restriction (Buchanan, Spencer, Goldsmith, & Catchpole, 2003; Schmidt, Moore, MacDougall-Shackleton, & MacDougall-Shackleton, 2013; Spencer et al., 2003).
Nevertheless, food restriction can also cause specific song and neural deficits not always seen in birds treated only with CORT. For example, song sparrows (Melsospiza melodia) that were either treated with CORT or food restricted both experienced a reduction in song complexity, but only food-restricted males were less accurate copying tutor song and had smaller volumes of the song-related brain nucleus RA (Schmidt, Moore, et al. 2013). This indicates that the influence of developmental stress on song may not necessarily be mediated by CORT per se, but by changing resource availability or resource allocation strategies of young birds. That is, birds that are deprived of nutrients early in life may have fewer substrates to allocate toward brain development (Welberg & Seckl, 2001), or may prioritize the development of certain systems when faced with limited nutrients (Schew & Ricklefs, 1998). These resource allocation strategies may, for instance, manifest as catch-up growth, which is a period of accelerated growth after a period of food restriction (Metcalfe & Monaghan, 2001). Catch-up growth may be beneficial in the short term by improving chances of survival, but there may be lifelong physiological debts incurred to paying the costs of this compensation (Metcalfe & Monaghan, 2001). Songbirds that are food restricted in early life often show slower growth rates, but typically these birds catch up to their control counterparts in adulthood once the stressor has been removed (Krause, Honarmand, Wetzel, & Naguib, 2009; Krause & Naguib, 2011; Kriengwatana, Wada, Macmillan, & MacDougall-Shackleton, 2013; Schmidt, MacDougall-Shackleton, & MacDougall-Shackleton, 2012; Spencer et al., 2003). Collectively, these studies suggest that glucocorticoid regulation is an important, but not the sole, mechanism by which developmental stress can have long-lasting impacts on birdsong. Glucocorticoid regulation may in fact be a component of resource allocation strategies that young birds employ when faced with stress during development (Wingfield et al., 1998).
The inconsistent effects of developmental stress on zebra finch song provide important clues about factors that can alter the impact of developmental stress on song. Specifically, the variety of developmental manipulations used across the studies to date (discussed in Kriengwatana et al., 2014), the relative importance of song in courtship displays, and relaxed sexual selection pressures due to domestication and variation among zebra finch colonies may contribute to these divergent findings. Increasing brood size and increasing foraging difficultly are two common methods of increasing developmental stress because of their ecological validity. However, it is difficult to identify the reasons why identical manipulations would yield different results (e.g., Brumm et al., 2009; Kriengwatana et al., 2014; Spencer et al., 2003; Zann & Cash, 2008) when researchers cannot quantify exactly how much stress is being applied to a nest and if all nestlings within a brood are experiencing the stressor equally. That is, it is logistically difficult to isolate zebra finch nestlings and strictly enforce a stressor if they are being fed by their parents and living in a brood. The fact that zebra finch song is part of a multimodal courtship display (i.e., females can see and hear males during courtship) suggests that their song may not be under as intense sexual selection as other species’ because song is one among many signals that zebra finch females use to assess potential mates (Bennett, Cuthill, Partridge, & Maier, 1996; Collins, Hubbard, & Houtman, 1994; Collins & ten Cate, 1996). In contrast to studies on zebra finches, data from free-living songbirds in which song clearly is and continues to be under intense sexual selection, such as European starlings (Sturnus vulgaris) and song sparrows (Melospiza melodia), have provided strong support for the developmental stress hypothesis (European starlings: Buchanan et al., 2003; Farrell, Weaver, An, & MacDougall-Shackleton, 2011; Spencer, Buchanan, Goldsmith, & Catchpole, 2004; song sparrows: MacDonald, Kempster, Zanette, & MacDougall-Shackleton, 2006; Schmidt, Moore, et al., 2013). Developmental stressors impair song learning and development of the song-control system in these species, and the size of song nucleus HVC is correlated with sexually selected components of song in free-living birds (starlings: Bernard, Eens, & Ball, 1996; song sparrows: Pfaff, Zanette, MacDougall-Shackleton, & MacDougall-Shackleton, 2007). Therefore, if song is a highly relevant indicator of fitness for a species, then we would expect that the mechanisms regulating song in that species are more susceptible to early environmental manipulations (Buchanan et al., 2013). Emphatically, developmental stress may not have the same consequences for fitness across all species or populations (i.e., colonies of domesticated birds) if song is not a primary metric by which females are evaluating potential mates.
Females and the Developmental Stress Hypothesis
As birdsong is the metric by which developmental stress has been assessed in most studies, little effort has been made to understand how stressors during development affect the receiver’s ability to perceive and respond to song. To our knowledge, there are no studies to date of the effects of developmental stress on a male’s ability to perceive and respond to songs during development or in adulthood (but for a manipulation of the early tutoring environment see Sturdy, Phillmore, Sartor, & Weisman, 2001). There are, however, a limited number of studies examining how developmental stress affects females’ responses to songs.
Most work on the effects of stress on females has been conducted with zebra finches. Data collected so far support the notion that females prefer the songs of control males to their stressed counterparts, and that female preferences may be altered by developmental stress (Riebel, Naguib, & Gil, 2009; Spencer et al., 2005). Female zebra finches prefer the songs of control males to previously stressed males, which suggests that developmental stress alters songs in a biologically relevant fashion (Spencer et al., 2005). In Riebel et al. (2009), female zebra finches raised in large broods (therefore presumed to have experienced more developmental stress) demonstrated equally strong preference for their tutor song as females from smaller broods. But, when given two songs from unfamiliar males singing a ‘short’ or ‘long’ song based on motif duration, females from small broods demonstrated stronger absolute preferences for one song type (i.e., there was no consistent directionality of the preference; Riebel et al., 2009). In similar studies, females were found to prefer males whose developmental background matched their own—in song preference tests and live interactive mate choice trials the females from small broods preferred males from small broods, and vice versa for large brood females (Holveck, Geberzahn, & Riebel, 2011; Holveck & Riebel, 2010). However, food restriction early in life did not affect female zebra finch preferences for song complexity when given song from unfamiliar singers, but did reduce overall activity during mate choice trials (Woodgate et al., 2011; Woodgate, Bennett, Leitner, Catchpole, & Buchanan, 2010). Overall, these studies indicate that developmental stress can have some influence over a female’s response toward song (i.e., less motor activity), but there is no compelling evidence to suggest that developmental stress alters a female’s preference toward song based on measures of song quality alone (i.e., complexity, motif duration). Be that as it may, studies that have found preference effects also have a potential confound—the similarity of the stimulus songs to songs a female would have been exposed to during the sensorimotor phases of vocal development are often not accounted for. Exposure to song in early life is a determining force to shaping female zebra finches’ song preferences (Lauay, Gerlach, Adkins-Regan, & DeVoogd, 2004; Riebel, 2000). A female raised in a large brood may acquire a preference for songs that share similar features to the songs of her development (i.e., similar to the song of her stressed father and/or developmentally stressed siblings). Therefore, rather than solely assessing if stress affects preferences for songs that vary in complexity, an additional consideration may be to assess preference for songs that vary features that sound more or less similar to the songs heard during the sensorimotor learning phase.
Apart from zebra finches, the only other species to date that has been studied with respect to the effects of developmental stress on female song preferences are song sparrows. Females that experienced food restriction or CORT treatment show reduced preferences for conspecific song (versus a heterospecific song) compared to control females (Schmidt, McCallum, MacDougall-Shackleton, & MacDougall-Shackleton, 2013). In addition, these stressed females showed patterns of neural activity in auditory forebrain areas (as measured by immunoreactivity of the immediate-early gene Zenk) that were not different when they listened to either conspecific or heterospecific song, while control females show significantly more immunoreactivity when listening to conspecific than heterospecific song (Schmidt, McCallum, et al., 2013). This study suggests that female preferences are condition dependent (Cotton, Small, & Pomiankowski, 2006) and may in part be caused by differences in neural activation in auditory forebrain regions in response to song (Schmidt, McCallum, et al., 2013). Unlike the zebra finches studies, all song sparrows in the Schmidt, McCallum, et al. (2013) study had the same exposure to tutor songs (a combination of live and tape tutors) in development, and all stimulus songs were from males whose repertoires were unfamiliar. Therefore, differences in early-tutoring environments are not likely the cause of the weaker preferences seen in song sparrows.
Even though female preference and mating decisions are swayed by the quality of male song (Gil & Gahr, 2002; Searcy, 1992), what exactly does a ‘good’ song advertise? Does song advertise direct benefits (e.g., increased parental feeding through superior foraging abilities), indirect benefits (e.g., good genes), or both? Moreover, what characteristics of song are most constrained by early developmental stress, and are they the same characteristics that females are using to evaluate prospective mates? Assessing preferences, and how developmental stress may alter them, should be tailored to each species due to ecological and life history characteristics that differ between them. In some species, specific content within a song is important for preference, such as sharing songs, singing a local dialect, or singing specific syllables (e.g., fast trills in canaries; Gil & Gahr, 2002). However, the reasons why females prefer these song characteristics could be established through different mechanisms (Searcy & Andersson, 1986), and therefore developmental stress may affect some species more selectively. For example, zebra finch mate preferences are strongly affected by parental imprinting such that zebra finches raised by Bengalese finches (Lonchura striata domestica) will almost exclusively display sexually in adulthood to Bengalese finches rather than conspecifics (ten Cate & Vos, 1999). Yet, female house finches (Carpodacus mexicanus) tutored by a foreign dialect, or in complete isolation, still preferred song from their local dialect in adulthood, despite no previous experience with it, which suggests some song preferences may be innate (Hernandez & MacDougall-Shackleton, 2004). Species for whom early auditory experiences are instrumental to shaping song preferences may be more susceptible to developmental stress than those whose preferences are less dependent on auditory experience.
Studies in wild bird populations generally support the developmental stress hypothesis that birdsong evolved as an honest signal of the developmental history of the singer. Attention is now turning toward understanding how developmental stress affects the perception of song. Currently, it appears that developmental stress may alter how females respond to songs, and preferences in some instances, but no tests so far have convincingly shown that these results are caused by developmental stress impairing mechanisms of perception. This, along with other questions (e.g., Does developmental stress selectively affect song perception? What are the neural bases for such perceptual impairments? And, if perceptual abilities were compromised, what would be the consequences to an individual’s fitness?), will need to be addressed in order to fully understand how early developmental factors can influence the coevolution of production and perception of increasingly elaborate sexually selected signals.
Developmental Stress and Correlated Cognitive Traits: Are the Best Singers Also the Smartest?
Early-life environments are fundamental to shaping an organism’s phenotype, exerting effects on a multitude of physiological and cognitive-behavioral traits (Metcalfe & Monaghan, 2001). In songbirds, physiological effects of developmental stress include altered growth rates, body size, organ mass, and immune and metabolic functioning (Kriengwatana et al., 2013; Verhulst, Holveck, & Riebel, 2006). Developmental stress can also affect other cognitive and behavioral processes in addition to affecting song, such as associative learning in zebra finches (Fisher, Nager, & Monaghan, 2006; Kriengwatana, Farrell, Aitken, Garcia, & MacDougall-Shackleton, in press) and spatial learning in Western scrub-jays (see the Developmental Stress and Spatial Cognition section for a detailed discussion; Pravosudov, Lavenex, & Omanska, 2005). Importantly, song characteristics appear to be correlated with a number of physiological and cognitive measures. For instance, song is correlated with immune function, body condition, endocrine function, survival, and fitness in song sparrows (Hasselquist, Bensch, & von Schantz, 1996; MacDougall-Shackleton et al., 2009; Pfaff et al., 2007; Schmidt et al., 2012; Schmidt, MacDougall-Shackleton, Soma, & MacDougall-Shackleton, 2014), inhibitory control in song sparrows (Boogert, Anderson, Peters, Searcy, & Nowicki, 2011), problem solving in zebra finches (Boogert, Giraldeau, & Lefebvre, 2008), and spatial learning in European starlings (Farrell et al., 2011). Consequently, these correlations suggest that if song is an honest indicator of early-life conditions (as posited by the developmental stress hypothesis), then song could also be an honest indicator of the quality of other cognitive functions that develop in parallel with song. In this section, we investigate the extent to which song is predictive of other cognitive functions, examine how developmental stress may explain the persistence of the relationships between song and other cognitive functions even after the stressor has been removed, and draw attention to the importance of understanding whether the effects of developmental stress on cognition have significant fitness consequences.
It is only recently that researchers began to test the relatively simple idea that the best singers are also the smartest, even though it seems logical to assume that the neural processing required for song learning may be correlated to other cognitive functions (Catchpole, 1996; Nowicki & Searcy, 2011). Numerous neural systems develop in tandem, and correlations can arise if they have overlapping critical sensitive periods and resource requirements (Buchanan et al., 2013; Spencer & MacDougall-Shackleton, 2011). For instance, the neural systems that regulate song learning and spatial memory (song-control system and hippocampus, respectively) are functionally independent in the developing zebra finch (Bailey, Wade, & Saldanha, 2009). Yet, these systems have developmental schedules that likely overlap (Brainard & Doupe, 2002; Clayton, 1996) and therefore could both be simultaneously affected by stressful environmental factors. If both systems are shaped by the same environmental factors (such as stressful rearing environments), this could result in them being correlated in adulthood despite their functional independence (Nowicki et al., 1998). Farrell et al. (2011) illustrate such an association: starlings that experienced a nutritional stressor in early development were impaired on a spatial memory task and scored lower on a measure of song quality. Moreover, the starlings that were better at the spatial foraging task also went on to sing more complex songs in their first breeding season (Figure 2).
Figure 2. Developmental stress affected both spatial and song abilities in starlings (figures modified with permission from Farrell et al., 2011). (A) An overhead view of the spatial foraging arena used in the spatial memory task. Birds were tested daily for 4 weeks with the same formation of 4 of 16 cups baited with mealworms. Cups were covered with tissue paper so birds had to peck through the paper to obtain the worm. (B) Performance as measured by number of incorrect cups searched across the 4-week testing phase of the spatial memory task. Controls (blue line) birds made significantly fewer errors than the food-restricted birds (orange line) across the 4-week testing period. (C) A male starling from the study singing in an aviary. (D) Average song bout length for both the control and food-restricted males from the study. Males raised in control conditions sang significantly longer song bouts in an undirected singing situation than males raised in a food-restricted condition. (E) Males that sang longer song bouts made fewer errors on the spatial memory task during the first 2 weeks of testing. Each point represents a male starling from the study coded by its early developmental condition (blue circle: control male, orange triangle: food-restricted male), but the regression line is based on all birds.
However, song is not always predictive of other cognitive traits, because for every positive correlation there have been an almost equal number of null, or even negative, correlations between song quality and other cognitive traits. In the aforementioned study with song sparrows (Boogert, Anderson, et al., 2011), there was no significant relationship between repertoire size and performance on a color association task or a reversal task. Templeton, Laland, and Boogert (2014) tested flocks of zebra finches on the same problem-solving task as Boogert et al. (2008) but did not replicate Boogert et al.’s results showing a positive relationship between song complexity and problem-solving performance in zebra finches tested in isolation. Specifically, Templeton et al.’s (2014) study did not find any relationship between song complexity of solvers and non-solvers, nor between song complexity and latency to solve the task among solvers. The researchers cite responsiveness to social isolation as the reason underlying the correlation previously reported by Boogert et al. (2008). A separate study in song sparrows found a negative relationship between spatial memory and song repertoire size (Sewall, Soha, Peters, & Nowicki, 2013), which the authors suggest could be the result of a trade-off between the development of song and spatial cognitive systems.
What might explain why song is predictive of other cognitive functions in some studies but not others? One explanation is that song correlates only with performance on particular cognitive tests. However, not all of the above outlined tests have been validated to assess specific cognitive processes (Thornton, Isden, & Madden, 2014). Alternatively, developmental history (i.e., the amount of developmental stress experienced) may contribute to differences in experience, motivation, and other factors that contribute to performance on cognitive tests (reviewed in Thornton & Lukas, 2012). Unfortunately, the developmental history of individuals is not always reported, making comparisons across studies rather difficult. Developmental history must be considered if we are to understand the importance of developmental stressors in organizing correlations among adult cognitive and behavioral traits—in this case, among song learning and other types of learning abilities. In light of these confounds, we strongly advise researchers interested in knowing how song is linked to other cognitive processes to use more rigorous testing and established experimental protocols (i.e., tests that have been validated to assess specific cognitive processes), and to always take note and report the developmental history of experimental subjects. To make the claim that song quality is predictive of other cognitive abilities, the onus must be placed on experimenters to demonstrate that these correlations are due to condition-dependent effects on the systems in question (Buchanan et al., 2013).
The relationship between song and other cognitive processes is predicted to be positive if the development of neural substrates mediating song and cognition overlap in time and if developmental stressors similarly affect song and cognition. Null or negative correlations between song and other cognitive traits could be explained if the neural substrates supporting a particular cognitive ability are developed at different times than the song-control system, or if the development of neural/physiological systems that maintain more essential functions than song are canalized, or buffered from developmental stressors. A third (and often implicit) assumption made when predicting the relationship between song and other cognitive functions is that they are, at least partly, mediated by common learning processes.
If song learning really does signal general learning abilities, this implies that there are common underlying processes for song and other forms of cognition, that is, a general intelligence factor in songbirds. General intelligence is a construct that captures cognitive performance across a series of tasks and suggests that performance on one task may be reflective of performance on other tasks (Deary, Penke, & Johnson, 2010). To date, there is evidence for general intelligence abilities in several species (reviewed in Boogert, Fawcett, & Lefebvre, 2011; Thornton & Lukas, 2012). Large-scale cognitive testing in birds is in its infancy, but we turn to recent studies on whether the elaborate bower-building displays (i.e., structures made of sticks and objects) of bowerbirds are reflective of a general intelligence factor (Isden, Panayi, Dingle, & Madden, 2013; Keagy, Savard, & Borgia, 2011a; Keagy, Savard, & Borgia, 2011b). Overall, there are few direct correlations between performances on different cognitive tasks in male satin bowerbirds (Ptilonorhynchus violaceus), but there is some evidence for a general intelligence factor, calculated based on shared covariance between the tests using principal components analysis (i.e., SB-g; Keagy et al., 2011b). However, in this species measures of song quality did not correlate with individual problem-solving abilities (Keagy et al., 2011b). In a congeneric species, spotted bowerbirds (Ptilonorhynchus nuchalis), one factor explains 44% of the covariance of test performance across a battery of tests unrelated to bower building (unlike tasks performed by Keagy, Savard, & Borgia, 2009, 2011a, 2011b). However, none of the individual tests or this aggregate score correlated with mating success (but see Isden et al., 2013). To date, the evidence from bowerbirds does not support the hypothesis that song quality is related to a general intelligence factor extracted from performance across various cognitive tests. Female bowerbirds appear to be making mating decisions on both measures of song and bower building in these species, which suggests that these traits are likely advertising different information (Candolin, 2003). Therefore, any general intelligence factors that are extracted from these studies likely reflect cognitive processes more or less independent of those necessary for song learning and performance.
Cognition and Fitness
Although there is good evidence that females of at least some songbird species preferentially choose males based on song (e.g., Hasselquist et al., 1996; Searcy, 1992), there is limited evidence that females choose males directly based on cognitive abilities (Boogert, Fawcett, et al., 2011). For example, female red-crossbills (Loxia curvirostra) observed males extracting seeds from pinecones and displayed a preference for the males that were faster at the task (Snowberg & Benkman, 2009), but female zebra finches showed no preference for males’ foraging technique (Boogert, Bui, Howarth, Giraldeau, & Lefebvre, 2010). Future research is warranted to determine whether females use song to assess a male’s cognitive abilities, or if they are also directly assessing other cognitive behaviors in mate choice situations. However, if a female chooses to mate with a “smarter” male it may not ultimately matter whether her decision was based directly on cognition or not, as long as she receives the fitness benefits (direct and/or indirect) from mating with a “smarter” male (Boogert, Fawcett, et al., 2011).
Song is a trait on which females appear to base mate choice decisions and this mate choice is hypothesized to result in fitness gains if that male also has greater cognitive abilities. However, rarely is the link between song and cognitive ability studied within the context of fitness. In the previous sections, we have reviewed the tenuous relationship between song and other cognitive domains. Most of the cognitive tests used are artificial ones designed by experimenters, making it difficult to determine whether performance on such tests is related to fitness in a natural setting. A behavior that is deemed “smart” by an experimenter may not yield an increase in overall fitness if: (a) the task is far removed from a biologically relevant context, (b) there are alternative strategies that are equally or more profitable (e.g., foraging versus scrounging), or (c) displaying such a behavior would not add, or would be counterproductive, to an animal’s reproductive success/fitness. Ultimately, “smart” behaviors evolve because they increase an individual’s survivability and/or reproductive success. And yet, how particular cognitive phenotypes benefit an animal’s fitness is a question that often falls outside the scope of most experiments (Healy, 2012).
Intelligence is assumed to be beneficial, but very few studies have found a direct link between cognition and fitness. In fact, researchers often do not consider the costs that may result from being smart. In one study that did so, the problem-solving abilities of over 400 great tits (Parus major) were related to their reproductive success (Cole, Morand-Ferron, Hinks & Quinn, 2012). Female tits briefly tested in captivity that were successful at a problem-solving task went on to have larger clutches and fledged more young. These fitness gains did not come at the expense of the mother’s own condition, but rather because these females had significantly smaller foraging areas. This implies that successful solvers were better able to exploit their habitat when foraging and therefore spent less time away from the nest, which in turn meant that they could engage in more nest-attentive behaviors that would increase the odds that young successfully fledged (Cole et al., 2012). But, successful solvers were also more likely to desert their nest after a nest disruption. Solvers in this population are known to have a heightened startle response and are less competitive in social situations (Cole & Quinn, 2012; Dunn, Cole, & Quinn, 2011); thus problem-solving appears to have both costs and benefits in this species.
Together, the above findings suggest that in this population of great tits there could be two alternative life-history strategies, with each strategy resulting in higher fitness benefits under particular circumstances. Solvers appear to be more reactive to nest disruptions and therefore could fledge fewer young compared to non-solvers in a season where nest disturbance events are high. Conversely, if food was not as abundant, solvers may fledge more young because they are able to exploit their habitats more efficiently than non-solvers. Therefore, cognitive ability may only be employed in situations where it is a rewarding strategy. If there is a genetic basis to these cognitive and personality phenotypes, then individuals may be under different selection pressures based upon ecological constraints (Svanbäck & Bolnick, 2007).
Developmental Stress and Spatial Cognition
In mammals, the association between developmental stress and deficits in spatial cognition in adulthood is well researched (Lupien, McEwen, Gunnar, & Heim, 2009; Vallée et al., 1999; Yang, Han, Cao, Li, & Xu, 2006). While studies of spatial cognition are prominent in the songbird literature, few have yet to manipulate developmental conditions and observe the effects on spatial cognition and the hippocampus. In this section, we review the few studies that have conducted such manipulations, remarking on a potential mechanism by which developmental stress could affect spatial cognition, and highlight future avenues of research.
For food-caching species, spatial cognition is a conspicuous trait that is closely linked to survival (Pravosudov & Lucas, 2001). Western scrub-jays (Aphelocoma claifornica) are prolific food-cachers—when food is plentiful, they store food in hidden locations and will retrieve these items in times of food scarcity. Pravosudov et al. (2005) hypothesized that stressful conditions in early development could induce cognitive deficits in scrub-jays, which would become evident when they performed tasks as adults that relied on spatial ability. This hypothesis was supported by their findings, as jays that experienced nutritional restriction in early life performed more poorly than control birds on tasks assessing cache recovery and spatial-association learning. Furthermore, when given conflicting spatial/color information to locate a food reward, the jays overwhelmingly preferred to solve the task based on the color information compared to birds that did not experience nutritional restriction. In the same birds, nutritional restriction impeded growth of the hippocampus, as food-restricted jays had smaller hippocampal volume and fewer hippocampal neurons, even though overall telencephalon volume and brain mass were unaffected. As memory for spatial location and memory for color appear to be mediated by separate mechanisms (Hampton & Shettleworth, 1996; Sherry & Vaccarino, 1989), Pravosudov et al.’s (2005) results reveal that the effects of stress in the brain are not uniform, and that some neural structures may be more sensitive to the effects of early nutritional stress than others.
For non-caching species, spatial cognition is involved in a variety of behaviors (e.g., migration, orientation, exploration; Shettleworth, 2009). In two non-food-caching species, manipulating diets early in life also had negative effects on performance in spatial memory tasks. Arnold, Ramsay, Donaldson, and Adam (2007) manipulated levels of taurine, an amino acid implicated in brain development and the regulation of the stress response (Engelmann, Landgraf, & Wotjak, 2003; Lapin, 2003), in nestling blue tits (Cyanistes caruleus). When tested as juveniles, blue tit females that had been supplemented with taurine showed a trend toward committing fewer errors on a spatial reference memory task. Similarly, starlings that were subjected to an unpredictable food supply during the juvenile phase committed more reference and working memory errors on a spatial foraging task compared to starlings raised on an ad libitum diet (Farrell et al., 2011; Figure 2B). Zebra finches subjected to nutritional stress before nutritional independence also performed more poorly in a hippocampus-dependent spatial memory task (Kriengwatana et al., in press). Although spatial performance was affected by early developmental treatments, neither of the aforementioned studies assessed the hippocampus or any other neural structures.
Developmental stress exists in many forms (e.g., brood enlargement, nutritional deficits, unpredictable environments, parasites, and infectious diseases), yet the resulting effects on the physiology of the song-control system and the hippocampus are similar: smaller volumes and fewer neurons. Therefore, the hippocampal differences in jays reported by Pravosudov et al. (2005) may not be due to a nutritional deficit per se, but rather the result of a physiological mechanism that, when stimulated by environmental stress, inhibits neural development. As discussed previously, a likely mechanism is the HPA axis, which regulates the physiological stress response involving glucocorticoids (CORT). Glucocorticoids may have short-term activational and long-term organizational effects on the hippocampus because this brain region is rich in two receptors (mineralocorticoid receptors, MR, and glucocorticoid receptors, GR) that regulate negative feedback and thus mediate the effects of glucocorticoids (Liu et al., 1997). Stressful early conditions increase circulating glucocorticoids and alter levels of glucocorticoid receptors in the hippocampus, which may subsequently lead to spatial memory deficits (Banerjee, Arterbery, Fergus, & Adkins-Regan, 2012; Hodgson et al., 2007; Pravosudov & Kitaysky, 2006). In zebra finches, offspring that were deprived of maternal care had a more exaggerated stress response to social isolation and fewer MR were observed across the brain, including within the hippocampus (Banerjee et al., 2012). Similarly, a selectively bred line of zebra finches that had high CORT in response to acute stress were found to have worse performance on a spatial memory task and fewer MR within the hippocampus (Hodgson et al., 2007). MR is thought to preserve neuronal integrity and excitatory tone within the hippocampus, and therefore a decrease in their number could compromise cognitive processes specific to the hippocampus (Joëls, Karst, DeRijk, & de Kloet, 2008; Joëls, 2008).
Food-caching birds provide a fruitful model for future work, as there are many established experimental protocols for assessing a variety of aspects of spatial memory and its relation to the hippocampus. Clayton and colleagues have studied the various ways scrub-jays (Aphelocoma coerulescens) and western scrub-jays cache their food and convincingly demonstrate that these birds integrate temporal, contextual, and social information in memory (Clayton, Dally, & Emery, 2007; Clayton & Dickinson, 1998). We know that developmental stress impairs hippocampus development and spatial memory in this species, but how might such stress affect temporal, semantic, and social memory? These and other cognitive processes, such as emotional processing and memory formation, may also depend on hippocampus function (Eichenbaum, 1996). In addition, while the volume of the hippocampus may reflect spatial performance, there could be additional aspects aside from volume that contribute to spatial and other forms of cognition. Hippocampal volume is a proxy of spatial cognition, as variables such as age, captivity, and seasonal effects can alter hippocampal volume (Roth, Brodin, Smulders, LaDage, & Pravosudov, 2010). Examining small-scale changes, such as the integration of newly generated neurons within the hippocampus, may be a more sensitive measure that reflects variation within spatial memory ability. Future research examining the effects of developmental stress should include variables that capture neuron integration, such as neuron proliferation, neuron type/size, glial counts/size, dendritic branching, and length of branching (Roth, Brodin, et al., 2010). Moreover, examining differences in gene expression for MR and GR receptors may be of importance as the hippocampus is an extrahypothalamic site for negative feedback of the HPA axis (Welberg & Seckl, 2001). Differences in these small-scale measures may not be reflected in the larger-scale measure of hippocampal volume and will further our understanding of how developmental stress may affect the brain. Developmental stress affects hippocampal development and spatial cognition, but future efforts are required both to understand the neural mechanisms behind these effects and to clarify how other memory systems may be affected.
Personality/Behavioral Syndromes
Behavioral syndromes can be defined as “a suite of correlated behaviors reflecting between-individual consistency in behavior across multiple (two or more) observations” (Sih & Bell, 2008, p. 231). Correlated behaviors should maintain a consistent and stable relationship, which is to say they should not change in the face of transient factors (e.g., motivation), but can change based on life history stages and social contexts (Groothuis & Carere, 2005; Schuett & Dall, 2009; van Oers, 2005). The definition of behavioral syndromes is inclusive and subsumes other similar, but not synonymous terms, such as coping styles, personality, and temperament (Sih & Bell, 2008; Stamps & Groothuis, 2010a). Consequently, we consider studies using any of the aforementioned terms as studies of behavioral syndromes.
The suite of correlated traits that comprise behavioral syndromes in songbirds is currently not well understood. So far, the most comprehensive studies of avian behavioral syndromes have been undertaken in great tits (Parus major). However, we must be cautious about assuming that these findings are applicable to other birds. In great tits, the terms reactive and proactive have been used to describe birds that exhibit slow exploration of novel environments and increased latency to investigate a novel object, and fast exploration of a novel environment and reduced latency to investigate a novel object, respectively (reviewed in Groothuis & Carere, 2005). Compared to reactive birds, proactive birds are consistently more aggressive, socially dominant, less behaviorally flexible, and secrete less CORT in response to social and restraint stress (Baugh et al., 2012; Carere, Drent, Privitera, Koolhaas, & Groothuis, 2005; Carere, Groothuis, Möstl, Daan, & Koolhaas, 2003; van Oers, Drent, de Goede, & van Noordwijk, 2004; Verbeek, Boon, & Drent, 1996; Verbeek, Drent, & Wiepkema, 1994). These different personality types can be influenced by genetic and nongenetic factors (Carere, Drent, Koolhaas, & Groothuis, 2005; Drent, van Oers, & van Noordwijk, 2003; Stamps & Groothuis, 2010b; van Oers et al., 2004). Studies on birds such as zebra finches and black-capped chickadees (Poecile atricapillus) also find consistent individual differences in exploratory behaviors (An, Kriengwatana, Newman, & MacDougall-Shackleton, 2011; Beauchamp, 2000; David, Auclair, & Cézilly, 2011; Krause & Naguib, 2011; Schuett & Dall, 2009). In zebra finches, selection for physiological responsiveness to stress is also associated with differences in exploration. Specifically, in zebra finch lines artificially selected for low and high responses to acute restraint stress, greater exploratory behavior was linked to higher CORT only in the low CORT line (Martins, Roberts, Giblin, Huxham, & Evans, 2007). While the work in great tits has been extremely influential for understanding avian behavioral syndromes, we must be careful when generalizing across species because relationships between traits may not exist or may be different in other species. For example, exploration and boldness were not correlated in zebra finches or in a non-songbird (Japanese quail; Coturnix japonica; Martins et al., 2007; Zimmer, Boogert, & Spencer, 2013), and learning an acoustic discrimination task was positively correlated with exploratory behavior in black-capped chickadees but not in great tits (Groothuis & Carere, 2005; Guillette, Reddon, Hurd, & Sturdy, 2009).
Few studies in birds have investigated the impact of developmental experiences on behavioral syndromes, although their importance to behavioral syndromes is gaining recognition (Groothuis & Trillmich, 2011; Stamps & Groothuis, 2010a, 2010b; Trillmich & Hudson, 2011). As explained in detail earlier, stressors during development can affect diverse physiological and behavioral traits and consequently may influence behavioral syndromes if they can affect the strength and direction of the correlation between these traits (Spencer & MacDougall-Shackleton, 2011). Data from the studies available do not yet clearly establish how developmental stress affects behavioral syndromes. Among others, one important variable that is inconsistent between studies is the timing of developmental stress and the time at which behaviors are assessed. The timing of stress during development is an important source of variation in offspring behavior, with both prenatal and postnatal stress (as well as the time within those stages) having potentially different behavioral outcomes (Boogert, Zimmer, & Spencer, 2013; Henriksen, Rettenbacher, & Groothuis, 2011; Krause et al., 2009; Kriengwatana, 2013; but see Zimmer et al., 2013). Furthermore, the time at which behaviors are assessed is also important because behavioral variation between individuals may change across the lifespan, thus making it difficult to detect covariation (Sih & Bell, 2008). Below we discuss separately the studies that investigate prenatal and postnatal stress.
Prenatal Stress
Prenatal stress is assumed to affect offspring behaviors through altering maternal steroid hormone deposition in eggs, decreasing maternal investment during egg formation, or affecting maternal care behaviors such as incubation. In a comprehensive review of prenatal stress in birds, Henriksen et al. (2011) noted that maternal stress (via CORT injections in the female or unpredictability of feeding) reduced offspring competitiveness, but that this result was not always observed if CORT was injected directly into the eggs. The effects of CORT injections into eggs on fearfulness and anxiety are mixed, and it is not clear whether these measures can be treated as measures of boldness and exploration, or whether these measures showed individual consistency and inter-individual variation and can thus be deemed as components of a behavioral syndrome (for a discussion regarding fearfulness as an aspect of behavioral syndromes see Cockrem, 2007). One study in Japanese quail that injected CORT into eggs and measured both boldness and exploration found that prenatal stress increased exploration but not boldness (Zimmer et al., 2013). Moreover, birds in this study that received both prenatal and postnatal stress treatments tended to be the most explorative and risk taking compared to birds that received pre- or postnatal stress treatments or control treatment. This suggests a cumulative effect of pre- and postnatal stress on measures of behavioral syndromes. Nevertheless, more studies are needed before generalizations can be made about the effects of prenatal stress on behavioral syndromes. In addition to manipulating CORT, future studies should also consider whether incubation temperature could alter offspring behavior, as previous work shows that it is able to affect a variety of physiological measures (Henriksen et al., 2011). In addition, it will be important to explore variation between species that produce altricial versus precocial young, as the physiological systems that develop prenatally in ovo will markedly differ. Recent work from our lab (H. Wada, unpublished) found that manipulations of incubation temperature in zebra finches (that produce altricial young) had very different effects than those reported for wood ducks (that produce precocial young; Durant, Hepp, Moore, Hopkins, & Hopkins, 2010). Thus, the distinction between pre- and postnatal manipulations will vary for species with different developmental schedules.
Postnatal Stress
Sources of postnatal stress include food availability, sibling competition, parental favoritism, and disease/parasitism. Investigations of the effect of early postnatal stress on components of behavioral syndromes are shown in Table 1. The strongest evidence that developmental stress can affect behavioral syndromes comes from a study that found that reducing food intake of great tit nestlings increased exploration and boldness (Carere, Drent, Koolhaas, et al., 2005). Importantly, food rationing increased aggression in great tits artificially selected to be fast explorers, indicating that postnatal developmental stress was able to alter the relationship between behavioral traits that are part of a well-established behavioral syndrome. However increased boldness and exploration were also observed in two other studies where birds may have experienced less developmental stress. Naguib, Flörcke, and van Oers (2011) found that great tits that experienced less sibling competition were faster to investigate novel environments and objects. Arnold et al. (2007) also found that blue tits (Cyanistes caeruleus) were bolder if they had been supplemented with taurine (an inhibitor of HPA axis activity; Engelmann et al., 2003) as nestlings. The discrepancy between the studies above may be due to the different manipulations used to alter developmental conditions. More research is needed to clarify how different stressors may produce different effects on personality traits.
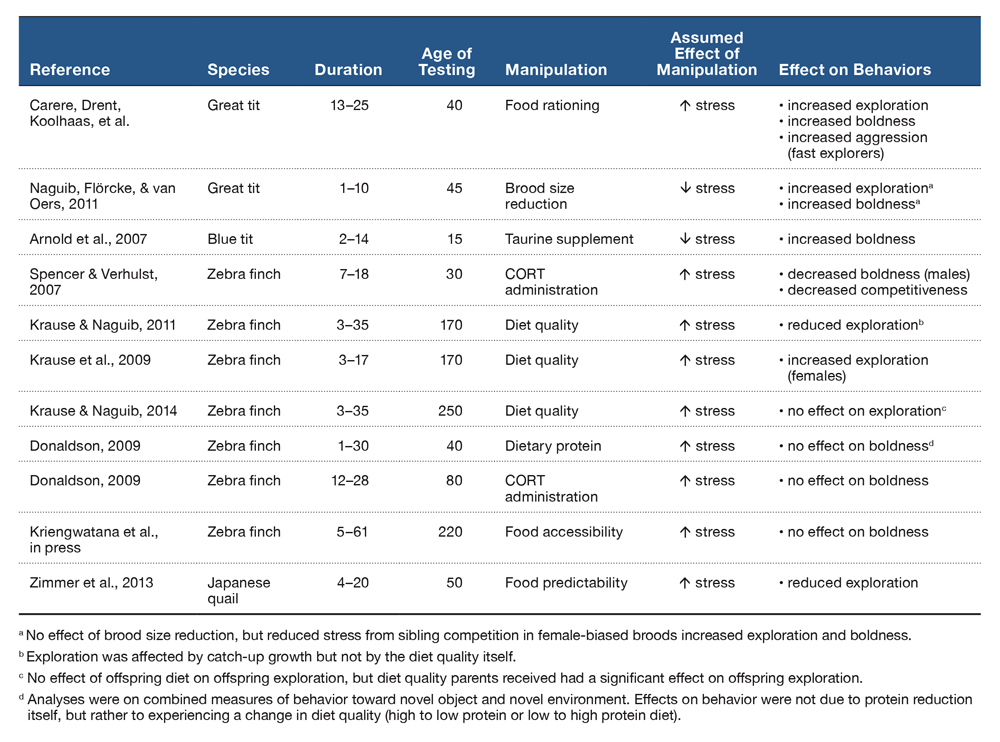
Table 1. A summary of studies of postnatal stress and the effects on components of behavioral syndromes. We distinguish between exploration and boldness as tests that measured behaviors in a novel environment, and toward a novel object, respectively. Values for the duration of treatment and approximate age of testing represent days post-hatch.
In zebra finches there are methodological, age, and sex-specific effects on the development of behavioral phenotypes. Administration of postnatal CORT has had contradictory effects, with one study reporting decreases in boldness (males only) and competitiveness (both sexes; CORT administered PHD 7–18: Spencer & Verhulst, 2007), and another reporting no change in boldness in either sex (CORT administered PHD 12–28; Donaldson, 2009). However, diet manipulations during a similar developmental time period generated different results. Krause et al. (2009) found that reducing diet quality increased exploration (females only; diet manipulation from PHD 1–17); however, the same manipulation for a longer duration had no effect on exploration on males or females (Krause & Naguib, 2014; PHD 3–35). Similarly, Donaldson (2009) found no effect of reducing dietary protein on boldness in either sex. Instead, Donaldson (2009) reported that inconsistency of treatment (e.g., a switch from high to low protein diets and vice versa) rather than the diet itself decreased boldness in both sexes, although this result was nonsignificant (p = 0.052). This suggests that environmental instability, rather than stressful early environments per se, may mediate the effects of developmental stress on personality. This hypothesis has received mixed support. In support, Krause and Naguib (2011) found that accelerated catch-up growth resulting from alleviation of nutritional stress was negatively correlated with exploration in males and females, but early nutritional stress itself did not affect exploration. In opposition, Kriengwatana et al. (in press) reported no effect of nutritional stress (via food accessibility) or constancy of nutritional conditions between PHD 5–61 on boldness in adult birds.
Despite some conflicting results, the studies above raise three important observations. First, findings that postnatal stress can decrease boldness (Spencer & Verhulst, 2007) and increase exploration (Krause et al., 2009) suggest that boldness and exploration may not be correlated in zebra finches (Martins et al., 2007). Alternatively, postnatal stress may not have affected both boldness and exploration because of the different type of stress experienced (i.e., CORT administration versus nutritional stress). An experiment that manipulates postnatal stress and measures both boldness and exploration in the same individuals is needed to evaluate these possibilities. Second, different types of stress during development interact with sex to differentially affect exploration and boldness in males and females. Males may be very sensitive to increased CORT during the first days post-hatch (Spencer & Verhulst, 2007), whereas both males and females may be similarly sensitive to unavailability of dietary protein before they reach nutritional independence (around PHD 35; Donaldson, 2009). Third, these results highlight that the effects of developmental stress on behavioral phenotypes is contingent on the age or life stage at which behaviors are assessed. Boldness or exploration are consistent in zebra finches if the tests are repeated within the same day, the next day, or the following week (David et al., 2011; Krause & Naguib, 2011; Schuett & Dall, 2009), yet over the long term these traits may change (Donaldson, 2009). The lack of correlations between behaviors at different life stages may reflect less behavioral variability of a species in general at a certain age, or be caused by testing for behaviors in contexts that do not sufficiently reveal underlying inter-individual variability (Sih & Bell, 2008).
In summary, both prenatal and postnatal developmental stress can alter behaviors that constitute behavioral syndromes, but further investigation is necessary to determine whether stress can change the correlations between traits. Further studies are also required to determine whether the influence of developmental stress on behavioral syndromes is limited to early life—the only study that manipulated stress after nutritional independence found that it had no effect on boldness (Kriengwatana et al., in press). Another aspect that warrants further investigation is how developmental stress differentially affects behavioral syndromes in males and females. Developmental stress may have sex-specific effects because different sexes may respond to stress differently according to their life history strategies, and already there is some evidence of developmental stress producing sex differences in boldness and/or exploration (e.g., Arnold et al., 2007; Donaldson, 2009; Spencer & Verhulst, 2007). As males seem to be more consistent in exploration and boldness compared to females (Donaldson, 2009; Schuett & Dall, 2009), this indicates that females may be more behaviorally plastic than males. Last, because developmental stress can have such diverse effects, it would be beneficial to assess the relationship of boldness, exploration, and aggression with other behaviors that may be affected by stress, such as begging rates, song, and learning ability (Arnold et al., 2007; Brust, Krüger, Naguib, & Krause, 2014; Carere, Drent, Koolhaas, et al., 2005; Garamszegi, Eens, & Török, 2008; Groothuis & Carere, 2005). Studies in this direction would address how developmental experiences, by altering behavioral tendencies, can affect behavioral plasticity.
Conclusion
Here we have reviewed the evidence to date for the developmental stress hypothesis and how we can extend its underlying principles to the study of other cognitive traits and behavioral measures. Cognitive and behavioral traits that are influenced by environmental conditions could be signaled through song quality, conveying information to listeners about how well an individual coped with stressful early-life conditions and/or their heritable developmental stability. Although there has been much borne out of the developmental stress hypothesis, there are still areas where current research falls short and there are areas where concentrated efforts are still needed.
The predictions of the developmental stress hypothesis have been supported in multiple species using a variety of manipulations. However, not all manipulations have yielded the same effects on song (reviewed in Spencer & MacDougall-Shackleton, 2011), and therefore more research is needed to understand the mechanisms by which developmental stress operates. As we alluded to throughout the review, CORT is a likely candidate by which stress affects the brain. Recent research has found that there are receptors for corticosterone in the song-control system (Suzuki, Matsunaga, Kobayashi, & Okanoya, 2011), and therefore corticosterone is a likely vehicle by which stress alters song learning. Still, many questions remain unanswered. For instance, how does CORT affect cellular processing, neuronal migration, or connectivity between neural circuits? And do these CORT-induced neural changes lead to observable behavioral changes in song? Another important factor to consider are sex-specific differences with regard to developmental stress. Although it is the male of the species that typically sings, how stress affects females’ perception, preference, and choice for male song is a crucial component of the evolutionary equation. A better understanding of what benefits are bestowed upon a female when she chooses a male will go a long way to understanding the evolution of this sexually selected cognitive trait.
We emphasize that most work to date regarding the correlation across cognitive traits and birdsong is equivocal. However, the field is still in its infancy and future studies should strive to use validated psychometric tests, adequate sample sizes, and knowledge about developmental history of its subjects. It is easier to assess cognitive abilities with localized neural structures, such as spatial cognition and the hippocampus. As reviewed above, there are many excellent songbird models that study hippocampal functioning that are rich sources for future studies linking song and with various aspects of memory. Designing future experiments around tasks that assess known cognitive processes and underlying neural structures is a necessary step to further our understanding between song and specific forms of cognition. For example, consider the arcopallium, a region homologous to the mammalian amygdala (Abellán, Legaz, Vernier, Rétaux, & Medina, 2009) that regulates fear learning and is sensitive to CORT (Brown, Woolston, & Frol, 2008; Cohen, 1975). Differences in the volume of the arcopallium between two black-capped chickadee populations could potentially explain the differences in problem-solving abilities and neophobia responses also observed between these two populations (Roth, Gallagher, LaDage, & Pravosudov, 2012; Roth, LaDage, & Pravosudov, 2010). Examining how developmental stress may affect the functioning of this area, and subsequent associative fear learning, could be one example of a future study assessing behavior with known neural correlates.
Although we have discussed personality and cognitive traits separately, they should not be thought of as independent of each other. It is apparent that early developmental conditions can shape an organism’s phenotype, but more work is needed to understand how such changes could give rise to persistent behavioral strategies across a variety of contexts. The timing of developmental stress, and the temporal overlap between periods when traits are most sensitive to environmental influences are key factors that could explain the relationship between personality and cognitive abilities.
In conclusion, to understand cognition and the relationship among various cognitive traits, it is imperative that we have knowledge of an individual’s developmental history. This is because developmental events, especially stressful ones, can have persistent effects on the function of various cognitive traits that carry over into adulthood. The developmental stress hypothesis provides a powerful framework to synthesize findings across the fields of developmental and cognitive research. While the hypothesis focuses on explaining variation within birdsong, its central tenets can be applied to other aspects of an individual’s condition, and the principle in general can be applied to other animals’ systems.
References
Abellán, A., Legaz, I., Vernier, B., Rétaux, S., & Medina, L. (2009). Olfactory and amygdalar structures of the chicken ventral pallium based on the combinatorial expression patterns of LIM and other developmental regulatory genes. The Journal of Comparative Neurology, 516(3), 166–186. doi:10.1002/cne.22102
An, Y. S., Kriengwatana, B., Newman, A. E., MacDougall-Shackleton, E. A., & MacDougall-Shackleton, S. A. (2011). Social rank, neophobia and observational learning in black-capped chickadees. Behaviour, 148(1), 55–69. doi:10.1163/000579510X545829
Andersen, S. L., & Teicher, M. H. (2008). Stress, sensitive periods and maturational events in adolescent depression. Trends in Neurosciences, 31(4), 183–191. doi:10.1016/j.tins.2008.01.004
Arnold, K. E., Ramsay, S. L., Donaldson, C., & Adam, A. (2007). Parental prey selection affects risk-taking behaviour and spatial learning in avian offspring. Proceedings of The Royal Society B: Biological Sciences, 274(1625), 2563–2569. doi:10.1098/rspb.2007.0687
Bailey, D. J., Wade, J., & Saldanha, C. J. (2009). Hippocampal lesions impair spatial memory performance, but not song—a developmental study of independent memory systems in the zebra finch. Developmental Neurobiology, 69(8), 491–504. doi:10.1002/dneu.20713
Banerjee, S. B., Arterbery, A. S., Fergus, D. J., & Adkins-Regan, E. (2012). Deprivation of maternal care has long-lasting consequences for the hypothalamic-pituitary-adrenal axis of zebra finches. Proceedings of The Royal Society B: Biological Sciences, 279(1729), 759–766. doi:10.1098/rspb.2011.1265
Baugh, A. T., Schaper, S. V., Hau, M., Cockrem, J. F., de Goede, P., & van Oers, K. (2012). Corticosterone responses differ between lines of great tits (Parus major) selected for divergent personalities. General and Comparative Endocrinology, 175(3), 488–494. doi:10.1016/j.ygcen.2011.12.012
Beauchamp, G. (2000). Individual differences in activity and exploration influence leadership in pairs of foraging zebra finches. Behaviour, 137, 301–314. doi:10.1163/156853900502097
Bennett, A. T. D., Cuthill, I. C., Partridge, J. C., & Maier, E. J. (1996). Ultraviolet vision and mate choice in zebra finches. Nature, 380(4), 433–435. doi:10.1038/380433a0
Bernard, D. J., Eens, M., & Ball, G. F. (1996). Age- and behavior-related variation in volumes of song control nuclei in male European starlings. Journal of Neurobiology, 30(3), 329–339.
doi:10.1002/(SICI)1097-4695(199607)30:3<329
::AID-NEU2>3.0.CO;2-6
Boogert, N. J., Anderson, R. C., Peters, S., Searcy, W. A., & Nowicki, S. (2011). Song repertoire size in male song sparrows correlates with detour reaching, but not with other cognitive measures. Animal Behaviour, 81(6), 1209–1216. doi:10.1016/j.anbehav.2011.03.004
Boogert, N. J., Bui, C., Howarth, K., Giraldeau, L.-A., & Lefebvre, L. (2010). Does foraging behaviour affect female mate preferences and pair formation in captive zebra finches? PloS One, 5(12), e14340. doi:10.1371
/journal.pone.0014340
Boogert, N. J., Fawcett, T. W., & Lefebvre, L. (2011). Mate choice for cognitive traits: A review of the evidence in nonhuman vertebrates. Behavioral Ecology, 22(3), 447–459. doi:10.1093/beheco/arq173
Boogert, N. J., Giraldeau, L.-A., & Lefebvre, L. (2008). Song complexity correlates with learning ability in zebra finch males. Animal Behaviour, 76(5), 1735–1741. doi:10.1016/j.anbehav.2008.08.009
Boogert, N. J., Zimmer, C., & Spencer, K. (2013). Pre- and post-natal stress have opposing effects on social information use. Biology Letters, 9(2), 20121088. doi:10.1098/rsbl.2012.1088
Brainard, M. S., & Doupe, A. J. (2002). What songbirds teach us about learning. Nature, 417(6886), 351–8. doi:10.1038/417351a
Brown, E. S., Woolston, D. J., & Frol, A. B. (2008). Amygdala volume in patients receiving chronic corticosteroid therapy. Biological Psychiatry, 63(7), 705–709. doi:10.1016/j.biopsych.2007.09.014
Brumm, H., Zollinger, S. A., & Slater, P. J. B. (2009). Developmental stress affects song learning but not song complexity and vocal amplitude in zebra finches. Behavioral Ecology and Sociobiology, 63(9), 1387–1395. doi:10.1007/s00265-009-0749-y
Brust, V., Krüger, O., Naguib, M., & Krause, E. T. (2014). Lifelong consequences of early nutritional conditions on learning performance in zebra finches (Taeniopygia guttata). Behavioural Processes, 103. doi:10.1016/j.beproc.2014.01.019
Buchanan, K. L., Grindstaff, J. L., & Pravosudov, V. V. (2013). Condition dependence, developmental plasticity, and cognition: Implications for ecology and evolution. Trends in Ecology & Evolution, 28(5), 290–296. doi:10.1016/j.tree.2013.02.004
Buchanan, K. L., Spencer, K., Goldsmith, A. R., & Catchpole, C. K. (2003). Song as an honest signal of past developmental stress in the European starling (Sturnus vulgaris). Proceedings of The Royal Society B: Biological Sciences, 270(1520), 1149–1156. doi:10.1098/rspb.2003.2330
Candolin, U. (2003). The use of multiple cues in mate choice. Biological Reviews of the Cambridge Philosophical Society, 78(4), 575–595. doi:10.1017/S1464793103006158
Carere, C., Drent, P., Koolhaas, J., & Groothuis, T. G. G. (2005). Epigenetic effects on personality traits: Early food provisioning and sibling competition. Behaviour, 142(9), 1329–1355. doi:10.1163/156853905774539328
Carere, C., Drent, P. J., Privitera, L., Koolhaas, J. M., & Groothuis, T. G. G. (2005). Personalities in great tits, Parus major: Stability and consistency. Animal Behaviour, 70(4), 795–805. doi:10.1016/j.anbehav.2005.01.003
Carere, C., Groothuis, T. G. G., Möstl, E., Daan, S., & Koolhaas, J. (2003). Fecal corticosteroids in a territorial bird selected for different personalities: Daily rhythm and the response to social stress. Hormones and Behavior, 43(5), 540–548. doi:10.1016/S0018-506X(03)00065-5
Catchpole, C. K. (1996). Song and female choice: Good genes and big brains? Trends in Ecology & Evolution, 11(9), 358–360. doi:10.1016/0169-5347(96)30042-6
Clayton, N. S. (1996). Development of food-storing and the hippocampus in juvenile marsh tits (Parus palustris). Behavioural Brain Research, 74(1–2), 153–159. doi:10.1016/0166-4328(95)00049-6
Clayton, N. S., Dally, J. M., & Emery, N. J. (2007). Social cognition by food-caching corvids. The western scrub-jay as a natural psychologist. Philosophical Transactions of the Royal Society of London. Series B, Biological Sciences, 362(1480), 507–522. doi:10.1098/rstb.2006.1992
Clayton, N. S., & Dickinson, A. (1998). Episodic-like memory during cache recovery by scrub jays. Nature, 395(6699), 272–274. doi:10.1038/26216
Cockrem, J. F. (2007). Stress, corticosterone responses and avian personalities. Journal of Ornithology, 148(S2), 169–178. doi:10.1007/s10336-007-0175-8
Cohen, D. H. (1975). Involvement of the avian amygdalar homologue (archistriatum posterior and mediale) in defensively conditioned heart rate change. The Journal of Comparative Neurology, 160(1), 13–35. doi:10.1002/cne.901600103
Cole, E. F., Morand-Ferron, J., Hinks, A. E. E., & Quinn, J. L. L. (2012). Cognitive ability influences reproductive life history variation in the wild. Current Biology, 22(19), 1808–1812. doi:10.1016/j.cub.2012.07.051
Cole, E. F., & Quinn, J. L. (2012). Personality and problem-solving performance explain competitive ability in the wild. Proceedings of The Royal Society B: Biological Sciences, 279(1731), 1168–1175. doi:10.1098/rspb.2011.1539
Collins, S. A., Hubbard, C., & Houtman, A. M. (1994). Female mate choice in the zebra finch—the effect of male beak color and male song. Behavioral Ecology and Sociobiology, 35(1), 21–25. doi:10.1007/BF00167055
Collins, S. A., & ten Cate, C. (1996). Does beak colour affect female preference in zebra finches? Animal Behaviour, 52(1), 105–112. doi:10.1006/anbe.1996.0156
Cotton, S., Small, J., & Pomiankowski, A. (2006). Sexual selection and condition-dependent mate preferences. Current Biology, 16(17), R755–R765. doi:10.1016/j.cub.2006.08.022
David, M., Auclair, Y., & Cézilly, F. (2011). Personality predicts social dominance in female zebra finches, Taeniopygia guttata, in a feeding context. Animal Behaviour, 81(1), 219–224. doi:10.1016/j.anbehav.2010.10.008
de Kogel, C. H., & Prijs, H. J. (1996). Effects of brood size maniuplations on sexual attractiveness of offspring in the zebra finch. Animal Behaviour, 51, 699–708. doi:10.1006/anbe.1996.0073
Deary, I. J., Penke, L., & Johnson, W. (2010). The neuroscience of human intelligence differences. Nature Reviews Neuroscience, 11(3), 201–11. doi:10.1038/nrn2793
Donaldson, C. (2009). Post-natal environmental effects on behaviour in the zebra finch (Taeniopygia guttata). (Doctoral dissertation, University of Glasgow, UK, 2009). Id: glathesis:2009-937. http://theses.gla.ac.uk/id/eprint/937
Drent, P. J., van Oers, K., & van Noordwijk, A. J. (2003). Realized heritability of personalities in the great tit (Parus major). Proceedings of The Royal Society B: Biological Sciences, 270(1510), 45–51. doi:10.1098/rspb.2002.2168
Dunn, J. C., Cole, E. F., & Quinn, J. L. (2011). Personality and parasites: Sex-dependent associations between avian malaria infection and multiple behavioural traits. Behavioral Ecology and Sociobiology, 65(7), 1459–1471. doi:10.1007/s00265-011-1156-8
Durant, S. E., Hepp, G. R., Moore, I. T., Hopkins, B. C., & Hopkins, W. A. (2010). Slight differences in incubation temperature affect early growth and stress endocrinology of wood duck (Aix sponsa) ducklings. The Journal of Experimental Biology, 213(1), 45–51. doi:10.1242/jeb.034488
Eichenbaum, H. (1996). Is the rodent hippocampus just for “place”? Current Opinion in Neurobiology, 6(2), 187–195. doi:10.1016/S0959-4388(96)80072-9
Engelmann, M., Landgraf, R., & Wotjak, C. T. (2003). Taurine regulates corticotropin secretion at the level of the supraoptic nucleus during stress in rats. Neuroscience Letters, 348(2), 120–122. doi:10.1016/S0304-3940(03)00741-9
Farrell, T. M., Weaver, K., An, Y.-S., & MacDougall-Shackleton, S. A. (2011). Song bout length is indicative of spatial learning in European starlings. Behavioral Ecology, 23(1), 101–111. doi:10.1093/beheco/arr162
Fisher, M. O., Nager, R. G., & Monaghan, P. (2006). Compensatory growth impairs adult cognitive performance. PLoS Biology, 4(8), e251. doi:10.1371/journal.pbio.0040251
Garamszegi, L. Z., Eens, M., & Török, J. (2008). Birds reveal their personality when singing. PloS One, 3(7), e2647. doi:10.1371/journal.pone.0002647
Gil, D., & Gahr, M. (2002). The honesty of bird song: Multiple constraints for multiple traits. Trends in Ecology & Evolution, 17(3), 133–141. doi:10.1016/S0169-5347(02)02410-2
Gil, D., Naguib, M., Riebel, K., Rutstein, A., & Gahr, M. (2006). Early condition, song learning , and the volume of song brain nuclei in the zebra finch (Taeniopygia guttata). Journal of Neurobiology, 66(14), 1602–1612. doi:10.1002/neu.20312
Groothuis, T. G. G., & Carere, C. (2005). Avian personalities: Characterization and epigenesis. Neuroscience and Biobehavioral Reviews, 29(1), 137–150. doi:10.1016/j.neubiorev.2004.06.010
Groothuis, T. G. G., & Trillmich, F. (2011). Unfolding personalities: The importance of studying ontogeny. Developmental Psychobiology, 53(6), 641–655. doi:10.1002/dev.20574
Guillette, L. M., Reddon, A. R., Hurd, P. L., & Sturdy, C. B. (2009). Exploration of a novel space is associated with individual differences in learning speed in black-capped chickadees, Poecile atricapillus. Behavioural Processes, 82(3), 265–70. doi:10.1016/j.beproc.2009.07.005
Hampton, R. R., & Shettleworth, S. J. (1996). Hippocampal lesions impair memory for location but not color in passerine birds. Behavioral Neuroscience, 110(4), 831–835. doi:10.1037/0735-7044.110.4.831
Hasselquist, D., Bensch, S., & von Schantz, T. (1996). Correlation between male song repertoire, extra-pair paternity and offspring survival in the great reed warbler. Nature, 381(6579), 229–232. doi:10.1038/381229a0
Healy, S. D. (2012). Animal cognition: The trade-off to being smart. Current Biology, 22(19), R840–R841. doi:10.1016/j.cub.2012.08.032
Heim, C., & Nemeroff, C. B. (2001). The role of childhood trauma in the neurobiology of mood and anxiety disorders: Preclinical and clinical studies. Biological Psychiatry, 49(12), 1023–1039. doi:10.1016/S0006-3223(01)01157-X
Heim, C., Shugart, M., Craighead, W. E., & Nemeroff, C. B. (2010). Neurobiological and psychiatric consequences of child abuse and neglect. Developmental Psychobiology, 52(7), 671–690. doi:10.1002/dev.20494
Henriksen, R., Rettenbacher, S., & Groothuis, T. G. G. (2011). Prenatal stress in birds: Pathways, effects, function and perspectives. Neuroscience and Biobehavioral Reviews, 35(7), 1484–1501. doi:10.1016/j.neubiorev.2011.04.010
Hernandez, A. M., & MacDougall-Shackleton, S. A. (2004). Effects of early song experience on song preferences and song control and auditory brain regions in female house finches (Carpodacus mexicanus). Journal of Neurobiology, 59(2), 247–258. doi:10.1002/neu.10312
Hodgson, Z. G., Meddle, S. L., Roberts, M. L., Buchanan, K. L., Evans, M. R., Metzdorf, R., et al. (2007). Spatial ability is impaired and hippocampal mineralocorticoid receptor mRNA expression reduced in zebra finches (Taeniopygia guttata) selected for acute high corticosterone response to stress. Proceedings of The Royal Society B: Biological Sciences, 274(1607), 239–245. doi:10.1098/rspb.2006.3704
Holveck, M.-J., Geberzahn, N., & Riebel, K. (2011). An experimental test of condition-dependent male and female mate choice in zebra finches. PloS One, 6(8), 1–10. doi:10.1371/journal.pone.0023974
Holveck, M.-J., & Riebel, K. (2010). Low-quality females prefer low-quality males when choosing a mate. Proceedings of The Royal Society B: Biological Sciences, 277(1678), 153–160. doi:10.1098/rspb.2009.1222
Holveck, M.-J., Vieira de Castro, A. C., Lachlan, R. F., ten Cate, C., & Riebel, K. (2008). Accuracy of song syntax learning and singing consistency signal early condition in zebra finches. Behavioral Ecology, 19(6), 1267–1281. doi:10.1093/beheco/arn078
Isden, J., Panayi, C., Dingle, C., & Madden, J. (2013). Performance in cognitive and problem-solving tasks in male spotted bowerbirds does not correlate with mating success. Animal Behaviour, 86(4), 829–838. doi:10.1016/j.anbehav.2013.07.024
Joëls, M. (2008). Functional actions of corticosteroids in the hippocampus. European Journal of Pharmacology, 583, 312–321. doi:10.1016/j.ejphar.2007.11.064
Joëls, M., Karst, H., DeRijk, R., & de Kloet, E. R. (2008). The coming out of the brain mineralocorticoid receptor. Trends in Neurosciences, 31(1), 1–7. doi:10.1016/j.tins.2007.10.005
Keagy, J., Savard, J.-F., & Borgia, G. (2009). Male satin bowerbird problem-solving ability predicts mating success. Animal Behaviour, 78(4), 809–817. doi:10.1016/j.anbehav.2009.07.011
Keagy, J., Savard, J.-F., & Borgia, G. (2011a). Cognitive ability and the evolution of multiple behavioral display traits. Behavioral Ecology, 23(2), 448–456. doi:10.1093/beheco/arr211
Keagy, J., Savard, J.-F., & Borgia, G. (2011b). Complex relationship between multiple measures of cognitive ability and male mating success in satin bowerbirds, Ptilonorhynchus violaceus. Animal Behaviour, 81(5), 1063–1070. doi:10.1016/j.anbehav.2011.02.018
Kitaysky, A., Kitaiskaia, E., Wingfield, J., & Piatt, J. (2001). Dietary restriction causes chronic elevation of corticosterone and enhances stress response in red-legged kittiwake chicks. Journal of Comparative Physiology B: Biochemical, Systemic, and Environmental Physiology, 171(8), 701–709. doi:10.1007/s003600100230
Krause, E. T., Honarmand, M., Wetzel, J., & Naguib, M. (2009). Early fasting is long lasting: Differences in early nutritional conditions reappear under stressful conditions in adult female zebra finches. PloS One, 4(3), e5015. doi:10.1371/journal.pone.0005015
Krause, E. T., & Naguib, M. (2011). Compensatory growth affects exploratory behaviour in zebra finches, Taeniopygia guttata. Animal Behaviour, 81(6), 1295–1300. doi:10.1016/j.anbehav.2011.03.021
Krause, E. T., & Naguib, M. (2014). Effects of parental and own early developmental conditions on the phenotype in zebra finches (Taeniopygia guttata). Evolutionary Ecology, 28(2), 263–275. doi:10.1007/s10682-013-9674-7
Kriengwatana, B. (2013). Timing of developmental stress and phenotypic plasticity: Effects of nutritional stress at different developmental periods on physiological and cognitive-behavioral traits in the zebra finch (Taeniopygia guttata). (Doctoral dissertation, University of Western Ontario, Canada, 2013). University of Western Ontario—Electronic Thesis and Dissertation Repository. Paper 1469. http://ir.lib.uwo.ca/etd/1469
Kriengwatana, B., Farrell, T. M., Aitken S. D. T., Garcia, L., & MacDougall-Shackleton, S. A. (2015). Early-life nutritional stress affects associative learning and spatial memory but not performance on a novel object task. Behaviour, 152(2), 195-218. doi:10.1163/1568539X-00003239
Kriengwatana, B., Wada, H., Macmillan, A., & MacDougall-Shackleton, S. A. (2013). Juvenile nutritional stress affects growth rate, adult organ mass, and innate immune function in zebra finches (Taeniopygia guttata). Physiological and Biochemical Zoology, 86(6), 769–781. doi:10.1086/673260
Kriengwatana, B., Wada, H., Schmidt, K. L., Taves, M. D., Soma, K. K., & MacDougall-Shackleton, S. A. (2014). Effects of nutritional stress during different developmental periods on song and the hypothalamic-pituitary-adrenal axis in zebra finches. Hormones and Behavior, 65(3), 285–293. doi:10.1016/j.yhbeh.2013.12.013
Lapin, I. P. (2003). Neurokynurenines (Neky) as common neurochemical links of stress and anxiety. Advances in Experimental Medicine and Biology Volume, 527, 121–125. doi:10.1007/978-1-4615-0135-0_14
Lauay, C., Gerlach, N. M., Adkins-Regan, E., & DeVoogd, T. J. (2004). Female zebra finches require early song exposure to prefer high-quality song as adults. Animal Behaviour, 68(6), 1249–1255. doi:10.1016/j.anbehav.2003.12.025
Liu, D., Diorio, J., Tannenbaum, B., Caldji, C., Francis, D., Freedman, A., et al. (1997). Maternal care, hippocampal glucocorticoid receptors, and hypothalamic-pituitary-adrenal responses to stress. Science, 277(5332), 1659–1662. doi:10.1126/science.277.5332.1659
Lupien, S. J., McEwen, B. S., Gunnar, M. R., & Heim, C. (2009). Effects of stress throughout the lifespan on the brain, behaviour and cognition. Nature Reviews. Neuroscience, 10(6), 434–445. doi:10.1038/nrn2639
MacDonald, I. F., Kempster, B., Zanette, L., & MacDougall-Shackleton, S. A. (2006). Early nutritional stress impairs development of a song-control brain region in both male and female juvenile song sparrows (Melospiza melodia) at the onset of song learning. Proceedings of The Royal Society B: Biological Sciences, 273(1600), 2559–2564. doi:10.1098/rspb.2006.3547
MacDougall-Shackleton, S. A, Dindia, L., Newman, A. E. M., Potvin, D. A., Stewart, K. A., & MacDougall-Shackleton, E. A. (2009). Stress, song and survival in sparrows. Biology Letters, 5(6), 746–748. doi:10.1098/rsbl.2009.0382
MacDougall-Shackleton, S., & Spencer, K. (2012). Developmental stress and birdsong: Current evidence and future directions. Journal of Ornithology, 153(S1), 105–117. doi:10.1007/s10336-011-0807-x
Martins, T. L. F., Roberts, M. L., Giblin, I., Huxham, R., & Evans, M. R. (2007). Speed of exploration and risk-taking behavior are linked to corticosterone titres in zebra finches. Hormones and Behavior, 52(4), 445–453. doi:10.1016/j.yhbeh.2007.06.007
Metcalfe, N. B., & Monaghan, P. (2001). Compensation for a bad start: Grow now, pay later? Trends in Ecology & Evolution, 16(5), 254–260. doi:10.1016/S0169-5347(01)02124-3
Monaghan, P. (2008). Early growth conditions, phenotypic development and environmental change. Philosophical Transactions of the Royal Society of London. Series B, Biological Sciences, 363(1497), 1635–1645. doi:10.1098/rstb.2007.0011
Naguib, M., Flörcke, C., & van Oers, K. (2011). Effects of social conditions during early development on stress response and personality traits in great tits (Parus major). Developmental Psychobiology, 53(6), 592–600. doi:10.1002/dev.20533
Nowicki, S., Hasselquist, D., Bensch, S., & Peters, S. (2000). Nestling growth and song repertoire size in great reed warblers: Evidence for song learning as an indicator mechanism in mate choice. Proceedings of The Royal Society B: Biological Sciences, 267(1460), 2419–2424. doi:10.1098/rspb.2000.1300
Nowicki, S., Peters, S., & Podos, J. (1998). Song learning, early nutrition and sexual selection in songbirds. Integrative and Comparative Biology, 38(1), 179–190. doi:10.1093/icb/38.1.179
Nowicki, S., & Searcy, W. A. (2011). Are better singers smarter? Behavioral Ecology, 22(1), 10–11. doi:10.1093/beheco/arq081
Pfaff, J. A., Zanette, L., MacDougall-Shackleton, S. A., & MacDougall-Shackleton, E. A. (2007). Song repertoire size varies with HVC volume and is indicative of male quality in song sparrows (Melospiza melodia). Proceedings of The Royal Society B: Biological Sciences, 274(1621), 2035–2040. doi:10.1098/rspb.2007.0170
Pravosudov, V. V., & Kitaysky, A. S. (2006). Effects of nutritional restrictions during post-hatching development on adrenocortical function in western scrub-jays (Aphelocoma californica). General and Comparative Endocrinology, 145(1), 25–31. doi:10.1016/j.ygcen.2005.06.011
Pravosudov, V. V., Lavenex, P., & Omanska, A. (2005). Nutritional deficits during early development affect hippocampal structure and spatial memory later in life. Behavioral Neuroscience, 119(5), 1368–1374. doi:10.1037/0735-7044.119.5.1368
Pravosudov, V. V., & Lucas, J. R. (2001). A dynamic model of short-term energy management in small food-caching and non-caching birds. Behavioral Ecology, 12(2), 207–218. doi:10.1093/beheco/12.2.207
Riebel, K. (2000). Early exposure leads to repeatable preferences for male song in female zebra finches. Proceedings of The Royal Society B: Biological Sciences, 267(1461), 2553–2558. doi:10.1098/rspb.2000.1320
Riebel, K., Naguib, M., & Gil, D. (2009). Experimental manipulation of the rearing environment influences adult female zebra finch song preferences. Animal Behaviour, 78(6), 1397–1404. doi:10.1016/j.anbehav.2009.09.011
Roth, T. C., Brodin, A., Smulders, T. V., LaDage, L. D., & Pravosudov, V. V. (2010). Is bigger always better? A critical appraisal of the use of volumetric analysis in the study of the hippocampus. Philosophical Transactions of the Royal Society B: Biological Sciences, 365(1542), 915–931. doi:10.1098/rstb.2009.0208
Roth, T. C., Gallagher, C. M., LaDage, L. D., & Pravosudov, V. V. (2012). Variation in brain regions associated with fear and learning in contrasting climates. Brain, Behavior and Evolution, 79(3), 181–190. doi:10.1159/000335421
Roth, T. C., LaDage, L. D., & Pravosudov, V. V. (2010). Learning capabilities enhanced in harsh environments: A common garden approach. Proceedings of The Royal Society B: Biological Sciences, 277(1697), 3187–3193. doi:10.1098/rspb.2010.0630
Schew, W. A., & Ricklefs, R. E. (1998). Developmental plasticity. In J. M. Starck & R. E. Ricklefs (Eds.), Avian Growth and Development: Evolution Within the Altricial-Percocial Spectrum (pp. 288–304). Oxford: Oxford University Press.
Schmidt, K. L., MacDougall-Shackleton, E. A., & MacDougall-Shackleton, S. A. (2012). Developmental stress has sex-specific effects on nestling growth and adult metabolic rates but no effect on adult body size or body composition in song sparrows. The Journal of Experimental Biology, 215(18), 3207–3217. doi:10.1242/jeb.068965
Schmidt, K. L., MacDougall-Shackleton, E. A., Soma, K. K., & MacDougall-Shackleton, S. A. (2014). Developmental programming of the HPA and HPG axes by early-life stress in male and female song sparrows. General and Comparative Endocrinology, 196, 72–80. doi:10.1016/j.ygcen.2013.11.014
Schmidt, K. L., McCallum, E. S., MacDougall-Shackleton, E. A., & MacDougall-Shackleton, S. A. (2013). Early-life stress affects the behavioural and neural response of female song sparrows to conspecific song. Animal Behaviour, 85(4), 825–837. doi:10.1016/j.anbehav.2013.01.029
Schmidt, K. L., Moore, S. D., MacDougall-Shackleton, E. A., & MacDougall-Shackleton, S. A. (2013). Early-life stress affects song complexity, song learning and volume of the brain nucleus RA in adult male song sparrows. Animal Behaviour, 86(1), 25–35. doi:10.1016/j.anbehav.2013.03.036
Schuett, W., & Dall, S. R. X. (2009). Sex differences, social context and personality in zebra finches, Taeniopygia guttata. Animal Behaviour, 77(5), 1041–1050. doi:10.1016/j.anbehav.2008.12.024
Searcy, W. A. (1992). Song repertoire and mate choice in birds. American Zoologist, 32(1), 71–80. doi:10.1093/icb/32.1.71
Searcy, W. A., & Andersson, M. (1986). Sexual selection and the evolution of song. Annual Review of Ecology, Evolution and Systematics, 17, 507–533. doi:10.1146/annurev.es.17.110186.002451
Sewall, K. B., Soha, J. A., Peters, S., & Nowicki, S. (2013). Potential trade-off between vocal ornamentation and spatial ability in a songbird. Biology Letters, 9. doi:10.1098/rsbl.2013.0344
Sherry, D. F., & Vaccarino, A. L. (1989). Hippocampus and memory for food caches in black-capped chickadees. Behavioral Neuroscience, 103(2), 308–318. doi:10.1037/0735-7044.103.2.308
Shettleworth, S. J. (2009). Cognition, Evolution, and Behavior (2nd ed.). New York: Oxford University Press.
Sih, A., & Bell, A. M. (2008). Insight for behavioral ecology from behavioral syndromes. Advances in the Study of Behavior, 38, 227–281. doi:10.1016/S0065-3454(08)00005-3
Snowberg, L. K., & Benkman, C. W. (2009). Mate choice based on a key ecological performance trait. Journal of Evolutionary Biology, 22(4), 762–769. doi:10.1111/j.1420-9101.2009.01699.x
Spencer, K., Buchanan, K., Goldsmith, A., & Catchpole, C. (2003). Song as an honest signal of developmental stress in the zebra finch (Taeniopygia guttata). Hormones and Behavior, 44(2), 132–139. doi:10.1016/S0018-506X(03)00124-7
Spencer, K., Buchanan, K. L., Goldsmith, A. R., & Catchpole, C. K. (2004). Developmental stress, social rank and song complexity in the European starling (Sturnus vulgaris). Proceedings of The Royal Society B: Biological Sciences, 271 Suppl, S121–S123. doi:10.1098/rsbl.2003.0122
Spencer, K., & MacDougall-Shackleton, S. A. (2011). Indicators of development as sexually selected traits: The developmental stress hypothesis in context. Behavioral Ecology, 22(1), 1–9. doi:10.1093/beheco/arq068
Spencer, K., & Verhulst, S. (2007). Delayed behavioral effects of postnatal exposure to corticosterone in the zebra finch (Taeniopygia guttata). Hormones and Behavior, 51(2), 273–280. doi:10.1016/j.yhbeh.2006.11.001
Spencer, K., Wimpenny, J. H., Buchanan, K. L., Lovell, P. G., Goldsmith, A. R., & Catchpole, C. K. (2005). Developmental stress affects the attractiveness of male song and female choice in the zebra finch (Taeniopygia guttata). Behavioral Ecology and Sociobiology, 58(4), 423–428. doi:10.1007/s00265-005-0927-5
Stamps, J. A., & Groothuis, T. G. G. (2010a). The development of animal personality: Relevance, concepts and perspectives. Biological Reviews of the Cambridge Philosophical Society, 85(2), 301–325. doi:10.1111/j.1469-185X.2009.00103.x
Stamps, J. A., & Groothuis, T. G. G. (2010b). Developmental perspectives on personality: Implications for ecological and evolutionary studies of individual differences. Philosophical Transactions of the Royal Society B: Biological Sciences, 365(1560), 4029–4041. doi:10.1098/rstb.2010.0218
Sturdy, C. B., Phillmore, L. S., Sartor, J. J., & Weisman, R. G. (2001). Reduced social contact causes auditory perceptual deficits in zebra finches, Taeniopygia guttata. Animal Behaviour, 62(6), 1207–1218. doi:10.1006/anbe.2001.1864
Suzuki, K., Matsunaga, E., Kobayashi, T., & Okanoya, K. (2011). Expression patterns of mineralocorticoid and glucocorticoid receptors in Bengalese finch (Lonchura striata var. domestica) brain suggest a relationship between stress hormones and song-system development. Neuroscience, 194, 72–83. doi:10.1016/j.neuroscience.2011.07.073
Svanbäck, R., & Bolnick, D. I. (2007). Intraspecific competition drives increased resource use diversity within a natural population. Proceedings of The Royal Society B: Biological Sciences, 274(1611), 839–844. doi:10.1098/rspb.2006.0198
Templeton, C. N., Laland, K. N., & Boogert, N. J. (2014). Does song complexity correlate with problem-solving performance in flocks of zebra finches? Animal Behaviour, 92, 63–71. doi:10.1016/j.anbehav.2014.03.019
ten Cate, C., & Vos, D. R. (1999). Sexual imprinting and evolutionary processes in birds: A reassessment. Advances in the Study of Behavior, 28, 1–31. doi:10.1016/S0065-3454(08)60214-4
Thornton, A., Isden, J., & Madden, J. R. (2014). Toward wild psychometrics: Linking individual cognitive differences to fitness. Behavioral Ecology, 25(6), 1299-1301, 1–3. doi:10.1093/beheco/aru095
Thornton, A., & Lukas, D. (2012). Individual variation in cognitive performance: Developmental and evolutionary perspectives. Philosophical Transactions of the Royal Society B: Biological Sciences, 367(1603), 2773–2783. doi:10.1098/rstb.2012.0214
Trillmich, F., & Hudson, R. (2011). The emergence of personality in animals: The need for a developmental approach. Developmental Psychobiology, 53(6), 505–509. doi:10.1002/dev.20573
Tschirren, B., Rutstein, A. N., Postma, E., Mariette, M., & Griffith, S. C. (2009). Short- and long-term consequences of early developmental conditions: A case study on wild and domesticated zebra finches. Journal of Evolutionary Biology, 22(2), 387–395. doi:10.1111/j.1420-9101.2008.01656.x
Vallée, M., MacCari, S., Dellu, F., Simon, H., Le Moal, M., & Mayo, W. (1999). Long-term effects of prenatal stress and postnatal handling on age-related glucocorticoid secretion and cognitive performance: A longitudinal study in the rat. The European Journal of Neuroscience, 11(8), 2906–2916. doi:10.1046/j.1460-9568.1999.00705.x
van Oers, K. (2005). Context dependence of personalities: Risk-taking behavior in a social and a nonsocial situation. Behavioral Ecology, 16(4), 716–723. doi:10.1093/beheco/ari045
van Oers, K., Drent, P. J., de Goede, P., & van Noordwijk, A. J. (2004). Realized heritability and repeatability of risk-taking behaviour in relation to avian personalities. Proceedings of The Royal Society B: Biological Sciences, 271(1534), 65–73. doi:10.1098/rspb.2003.2518
Verbeek, M., Boon, A., & Drent, P. J. (1996). Exploration, aggressive behaviour and dominance in pair-wise confrontations of juvenile male great tits. Behaviour, 133, 945–963. doi:10.1163/156853996X00314
Verbeek, M. E. M., Drent, P. J., & Wiepkema, P. R. (1994). Consistent individual differences in early exploratory behaviour of male great tits. Animal Behaviour, 48(5), 1113–1121. doi:10.1006/anbe.1994.1344
Verhulst, S., Holveck, M.-J., & Riebel, K. (2006). Long-term effects of manipulated natal brood size on metabolic rate in zebra finches. Biology Letters, 2(3), 478–480. doi:10.1098/rsbl.2006.0496
Welberg, L. A. M., & Seckl, J. R. (2001). Prenatal stress, glucocorticoids and the programming of the brain. Journal of Neuroendocrinology, 13(2), 113–128. doi:10.1111/j.1365-2826.2001.00601.x
Wingfield, J. C., Maney, D. L., Breuner, C. W., Jacobs, J. D., Lynn, S., Ramenofsky, M., & Richardson, R. D. (1998). Ecological bases of hormone–behavior interactions: The “emergency life history stage.” Integrative and Comparative Biology, 38(1), 191–206. doi:10.1093/icb/38.1.191
Woodgate, J. L., Bennett, A. T. D., Leitner, S., Catchpole, C. K., & Buchanan, K. L. (2010). Developmental stress and female mate choice behaviour in the zebra finch. Animal Behaviour, 79(6), 1381–1390. doi:10.1016/j.anbehav.2010.03.018
Woodgate, J. L., Leitner, S., Catchpole, C. K., Berg, M. L., Bennett, A. T. D., & Buchanan, K. L. (2011). Developmental stressors that impair song learning in males do not appear to affect female preferences for song complexity in the zebra finch. Behavioral Ecology, 22(3), 566–573. doi:10.1093/beheco/arr006
Yang, J., Han, H., Cao, J., Li, L., & Xu, L. (2006). Prenatal stress modifies hippocampal synaptic plasticity and spatial learning in young rat offspring. Hippocampus, 436, 431–436. doi:10.1002/hipo.20181
Zann, R., & Cash, E. (2008). Developmental stress impairs song complexity but not learning accuracy in non-domesticated zebra finches (Taeniopygia guttata). Behavioral Ecology and Sociobiology, 62(3), 391–400. doi:10.1007/s00265-007-0467-2
Zimmer, C., Boogert, N. J., & Spencer, K. (2013). Developmental programming: Cumulative effects of increased pre-hatching corticosterone levels and post-hatching unpredictable food availability on physiology and behaviour in adulthood. Hormones and Behavior, 64(3), 494–500. doi:10.1016/j.yhbeh.2013.07.002