What hummingbirds can tell us about cognition in the wild
Susan D. Healy,
University of St. Andrews, St. Andrews, Fife, UK
T. Andrew Hurly,
University of Lethbridge, Lethbridge, Alberta, Canada
Reading Options:
PDF | Add to Endnote | Kindle | eBook
Abstract
Here we review around 20 years of experimental data that we have collected during tests of cognitive abilities of free-living, wild rufous hummingbirds Selasphorus rufus at their breeding grounds in southwestern Alberta. Because these birds are readily trained to feed from artificial flowers they have proved a useful system for testing cognitive abilities of an animal outside the box wherein animal cognitive abilities are so often tested in the laboratory. And, although these data all come from a single species in a single location, the long-term aim of this work is to make a contribution to our understanding of the evolution of cognitive abilities, by examining the relationship between the ecological demands these birds face and their cognitive abilities. Testing predictions based on our knowledge of their ecology we have found that, while these birds aggressively defend a territory and display to females during the time we train and test them, they can learn and remember the locations of rewarded flowers, what those flowers look like, and when they are likely to contain food. Small-brained though they may be, these 3g hummingbirds appear to have cognitive capabilities that are not only well matched to their ecological demands, they are in at least some instances better (more capacious) than those of animals tested in the laboratory.
Keywords: hummingbird; field research; spatial orientation; timing; choice
Acknowledgements: We thank Rachael Marshall for helpful comments on an earlier version of manuscript, NSERC for funding T.A.H. and all of the cabin team for their contributions to this work. Corresponding author: susan.healy@st-andrews.ac.uk All previously published figures and photographs are used with permission of the authors.
Around 15 years have passed since the publication of Animal Cognition in Nature (Balda, Pepperberg, & Kamil, 1998). Ironically, this was a volume that did not, in fact, actually contain any chapters examining the cognitive abilities of animals in the wild. It did, however, contain descriptions of work on wild animals trained and tested under laboratory conditions and seemed to herald a major expansion of work on comparative cognition to encompass a much wider range of species than previously tested. A decade and a half later, however, it is not clear that that promise is being realised. For example, food storing, once a model for examining questions of the evolution of cognition and possibly the wildest of all the examples discussed in Balda et al. (1998), is now much less of a focus (e.g., Biegler, McGregor, Krebs, & Healy, 2001; Hampton & Shettleworth, 1996; Sherry & Vaccarino, 1989; but see Feeney, Roberts, & Sherry, 2009; Freas, LaDage, Roth, & Pravosudov, 2012). Food storing did, however, lead to perhaps the greatest recent flurry of excitement and effort in comparative cognition (Clayton & Dickinson, 1999): the examination of cognitive abilities in corvids. Subsequent work is now ranging from examination of episodic-like memory in a number of species including rats (Babb & Crystal, 2005), magpies Pica pica (Zinkivskay, Nazir, & Smulders, 2009), chickadees Poecile atricapillus (Feeney, et al., 2009), hummingbirds (Henderson, Hurly, Bateson, & Healy, 2006a), and meadow voles Microtus pennsylvanicus (Ferkin, Combs, Delbarco-Trillo, Pierce, & Franklin, 2008) to examination of problem-solving in a variety of contexts, typically by corvids but not always (Auersperg, Huber, & Gajdon, 2011; Dally, Emery, & Clayton, 2010; Schmidt, Scheid, Kotrschal, Bugnyar, & Schloegl, 2011; Taylor, Elliffe, Hunt, & Gray, 2010; Teschke & Tebbich, 2011; Weir, Chappell, & Kacelnik, 2009).
In fact, much of comparative cognition can be comfortably addressed in the laboratory, even when wild animals are tested. This may help to explain why there continues to be very little examination of cognitive abilities of animals in the wild, in what might be considered to be the real world. That world is one in which animals are faced daily with getting food, finding mates, avoiding predation, and this is where selection acts on cognitive abilities, perhaps favouring animals that are generally smart or, alternatively, favouring animals that are good at solving particular problems. The questions, then, differ slightly from those asked of animals in a laboratory i.e., not just what animals can do but what and how do they put those abilities to work when the test itself does not occupy much of their day. It is possible that we will find that animals’ cognitive abilities in the field differ little or not at all from those we see in the laboratory. For example, the use of food deprivation in the laboratory to motivate animals to perform a test may resemble the state in which many wild animals find themselves i.e., often hungry and very willing to work for reliable food rewards. On the other hand, having to watch out for predators or competitors may mean that animals attend to experimental features differently than if they were to be tested in the field or the spatial scale over which testing occurs (Figure 1). Natural conditions might also lead to different cue use or different cue weighting than we see when animals are tested in boxes, arenas or (relatively) small rooms in the laboratory.
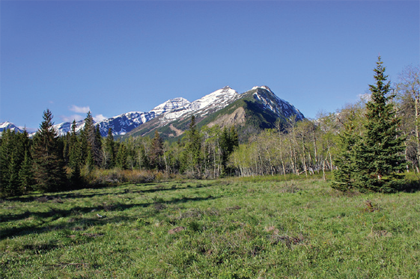
Figure 1. A photo showing the landscape in which we train and test our hummingbirds. Birds typically defend territories that contain both open fields and some wooded areas. In this photo, one bird defended a territory at the far end of the field and a second male defended a territory around the location at which the photograph was taken. Photo by T. A. Hurly.
Going out into the field to test cognitive abilities certainly shares problems with laboratory tests, not least of which is being sure that the animal ‘answers’ the question experimenters think they are asking. If an animal fails to respond in an experiment, for example, it is frequently unclear whether this is because the animal is not motivated to respond or does not ‘know’ how to respond. Only when the animal does make a response that seems vaguely appropriate can we begin to measure its performance. Even then, variation in its performance may be due to motivation rather than to cognitive ability per se. In the field the animal may be distracted mid-test or simply fail to return to the test after failing to find a reward. A second major issue that has arisen with recent tests of cognitive ability in the wild is with the ‘unit of measurement’ for cognitive ability, especially when problem solving is that measure. Thus far, we are not aware of a general consensus as to what constitutes a problem or what makes one problem more difficult than another. For example, currently, manipulation of physical material to retrieve food from manmade devices is considered by some to require ‘complex’ cognition although an apparently similar manipulation of materials to build a nest, however complex, is not (Seed & Byrne, 2010; but see Muth & Healy, 2011; Walsh, Hansell, & Healy, 2010; Walsh, Hansell, Borello, & Healy, 2011; van Casteren, Sellers, Thorpe, Coward, Crompton, Myatt, & Ennos, 2012). Is a problem considered more difficult if it has more steps to the solution, even if each step is ‘easy’, or is a problem more difficult if it is more novel, either in appearance or in its solution? It would seem that there is a problem in using problem solving as a measure for cognitive abilities in animals. And if we have no measure that is readily quantifiable, then it will not be possible to determine the causes or consequences of variation in that measure, within or across species.
It will come as no surprise that we have not attempted to examine problem solving in our work examining the cognitive abilities of rufous hummingbirds, trained and tested in the wild at our field site in the eastern Rocky Mountains, Alberta, Canada. At least, we have not looked at their ability to manipulate tools or to solve problems of the kinds that crows and others are now being set. Rather we have required our birds to learn to feed from all manner of devices (Figure 2), which they have invariably been very quick to do, typically learning within a couple of hours where to insert their tongue to receive sugar solution (sucrose). Although some might say that speed of learning in itself indicates cognitive ability (e.g., Boogert, Fawcett, & Lefebvre, 2011; Keagy, Savard, & Borgia, 2012), the fact that these birds learn so readily has for us largely meant that they are a useful species for examining cognition in the field: animals that took 100’s or 1000’s of trials to learn how to solve a task would have led us to look for other species. Here we review our work with two aims in mind: (1) to show that basing an experimental framework on knowing the ecology of a species can lead to a useful understanding of that species’ cognitive abilities, and, (2) in light of the paucity of work done in the wild, we want to use our work on rufous hummingbirds as a case study to show what is possible to do in the messiness of the field, where our control over the animal’s behaviour and experience is compromised. We would hope to show that such a pursuit can be both fruitful and that by doing so we add usefully to our understanding of cognition acquired from laboratory experiments. By so doing we would hope to encourage others similarly to go out into the field to examine cognition in other species. If we want to understand the evolution of cognitive abilities, especially in the vertebrates, the answers will not come from work on a single species, irrespective of the depth of enquiry.
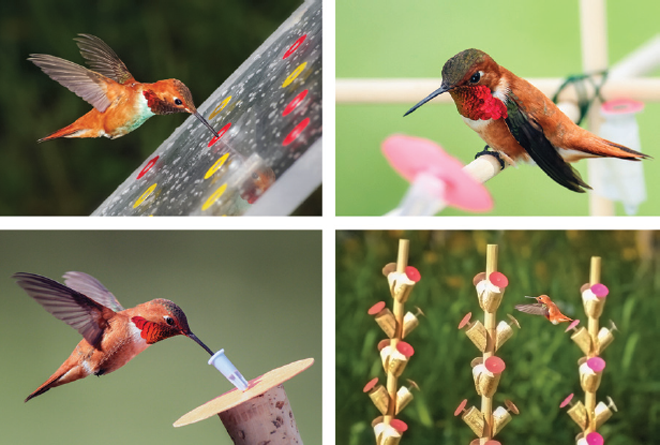
Figure 2. Four examples of the kind of feeding device to which the birds can be readily trained. With these ‘flowers’ we can vary the quantity of sucrose, the number of flowers, their spatial proximity and their visual features. The photograph at the top left is of a board of the kind we use in the context-dependent experiments. The next two photographs show birds about to and feeding from our most commonly-used flower type, a cardboard disc with a central well formed from a syringe tip or cap. The bottom right photograph shows a hummingbird choosing florets on artificial inflorescences. Photos by T. A. Hurly.
Interested as we are in comparative cognition, we have two significant reasons for attempting to determine the cognitive abilities in a single species, specifically rufous hummingbirds, in the wild. Firstly, these birds are logistically amenable to testing. As described elsewhere (e.g., Healy & Hurly, 2003, 2004), the males (the focus of our efforts) are strongly territorial, excluding conspecifics from feeding and thus from being trained to use our experimental equipment, they can be readily marked for individual identification and they feed every 10-15 minutes throughout the day for the duration of the breeding season (Figure 3). Although one might say that cognition is studied in the laboratory for logistic reasons such as experimental and experiential control, the choice of a species to test in the wild is, at this relatively early stage of such work, crucial to success. For example, the fact that our hummingbirds feed every 10-15 minutes means that we can collect a useful amount of data within a day and across our six-week field season. Choosing to work with animals that feed once a day or less often, would lead to major issues with training animals and collecting enough data, without each study taking a lifetime!
Equally key to the success of the endeavour is that the behaviour and ecology of these birds is such that we can readily formulate predictions as to the nature of the birds’ cognitive abilities from our observations of the birds’ foraging behaviour. Rather than using a rather arbitrary task to examine their cognitive abilities, we can attempt to test those abilities we would expect might have been favoured in these particular animals. Foraging behaviour in the male rufous hummingbirds, at least, typically consists of a male flying approximately every 10 minutes from a conspicuous perch in his territory to feed (from flowers or our feeders; Figure 4) for a handful of seconds before returning to his perch. The intervening time before his next foraging bout can be filled with a considerable activity as he is constantly on the lookout for conspecific males and females. Territorial males display to conspecific rivals by the flashing of their bright orange gorget (throat) feathers. If this does not deter an intruder, it will be chased off at high speed. Females are also chased, especially off the feeders, but they tend to move to a position near the ground while the male performs several display flights. These consist of the male flying up (some 15m) and then flying steeply downwards before pulling out of his dive just above the head of the female, flying a short upward sweep and ending with a waggle (a short series of oscillations in the vertical plane). He then either repeats this manoeuvre several times or flies to the female and performs a shuttle-flight – a series of short zig-zag buzzing flights in front of her. The aim of this game is to persuade the female to mate (Hurly, Scott, & Healy, 2001). Although we have not measured the energy expenditure of the males’ various flight acrobatics, it appears that they would be energetically expensive (Clark, 2009). Indeed, males tend to visit the feeder (flowers) within a few minutes of such displays, although their visits are still no longer than a few seconds. Our very first speculation with regard to their cognitive abilities, then, was that this small (about 3g) nectarivore, defending several hundred (or more) flowers and feeding about every ten minutes for a few seconds only, might benefit from remembering which flowers he had recently visited (Healy & Hurly 2001). Not only would he save time and energy by remembering where they were, it should be useful to remember whether he had emptied the flower(s), or not. A bird that could do this would return to territory defence and mate attraction more quickly having expended less energy.
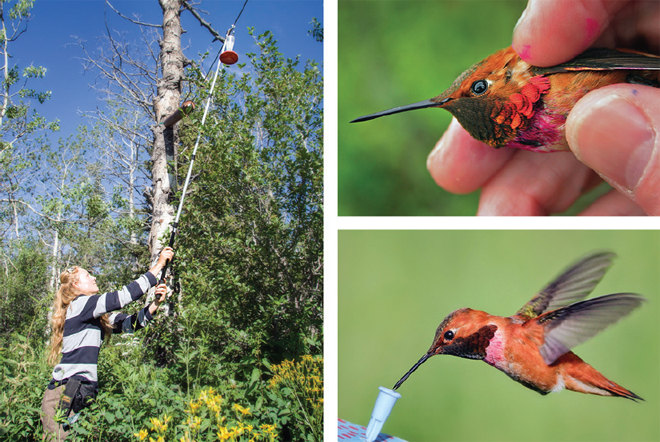
Figure 3. Photographs showing the elevated feeder (to deter bears) being lowered during pre-training (left), a newly marked bird in the hand (top right) and a marked bird feeding during an experiment (bottom right). Photos by T. A. Hurly.
The success of our very first, speculative experiment set the scene for most of the experiments that have followed. In that first experiment, we presented rufous hummingbirds with an open-field analogue of a radial-arm maze: an array of artificial flowers, some of which contained a small amount of sucrose solution (Healy & Hurly, 1995). The flowers were coloured cardboard discs approximately 6cm in diameter, each glued to the end of a wooden stake 60cm tall (Figures 2 and 5). They were arranged in a rough circle with about 70cm between neighbouring flowers. For this experiment, the flowers held 40μl, an amount that meant the birds should visit and drink all of the contents of about four of the eight flowers. We presented the birds with two versions of this delayed-non-matching-to-sample task: in one version, all eight flowers contained reward but we allowed birds to visit only up to four flowers on their first visit to the array and in the second version, all eight stakes were presented but only four bore flowers. For both versions, then, birds visited and emptied up to four flowers of their contents. On their return to the array, after intervals ranging from five minutes up to an hour, all eight flowers were present but only the flowers that had not been visited in the first phase of the trial contained food. As predicted, the birds were much more likely to visit flowers they had not recently emptied. A follow-up experiment showed that birds were also more likely to visit the flowers that had been present when they first visited the array but from which they did not drink (Hurly, 1996; see also Henderson, Hurly, & Healy, 2001). Although memory for perhaps as many eight flowers is not in the ball park of the number of flowers thought to make up the territory of these birds (perhaps a couple of thousand), the birds could remember not just where the flowers were but that they had emptied them.
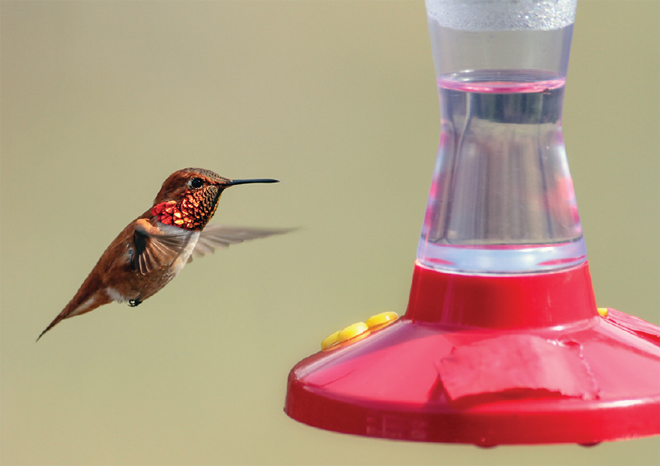
Figure 4. Once they arrive at our field site, the males establish feeding territories centred around feeders we have hung along the valley a week or two before they arrive. Typically the feeders contain 14% sucrose, which is much weaker than the nectar provided by the flowers from which the birds would normally feed. Photo by T. A Hurly.
That the birds could remember something about a flower’s contents was confirmed by an experiment in which we were actually aiming to address the role that flower colour played in the birds’ ability to learn which flowers to visit. We expected that the birds would pay attention to the colour of the flowers both because, like us, birds have well-developed colour vision and there is much anecdotal evidence that hummingbirds are attracted to red objects. There was also speculation that this predilection for red had lead to the propensity for the Californian flora, which lie on the migratory path of these birds, to produce red flowers. In that experiment we presented the birds with four, individually coloured flowers, only one of which contained sucrose solution and too much for a bird to consume in one visit. Once a bird had found the rewarded flower and then left after drinking as much as he wished, we emptied the flower and switched it with one of the other flowers in the array. When the bird returned, he was more likely to go to the flower that was in the location of the flower he had most recently fed from, rather than to the flower with the colour of the earlier, rewarded flower (Hurly & Healy, 1996; Miller & Miller, 1971; Miller, Tamm, Sutherland, & Gass, 1985). Consideration of the nature of the birds’ ecology helps to explain why these bird seemed to ignore the colour cue provided by the flower: in a field of flowers of the same species, colour does not help the bird determine which flowers will be rewarding. Colour might, however, be used to find flowers in unfamiliar places, such as along a migratory route and red may well be more conspicuous against a background of browns and greens that make up a western North American mountain range. It has also been argued elsewhere that the ubiquity of red flowers along the migration route of the rufous has less to do with attracting hummingbirds than making flowers inconspicuous to insects, whose vision is poorer at longer wavelengths (Altshuler, 2003; Briscoe & Chittka, 2001; Raven, 1972).
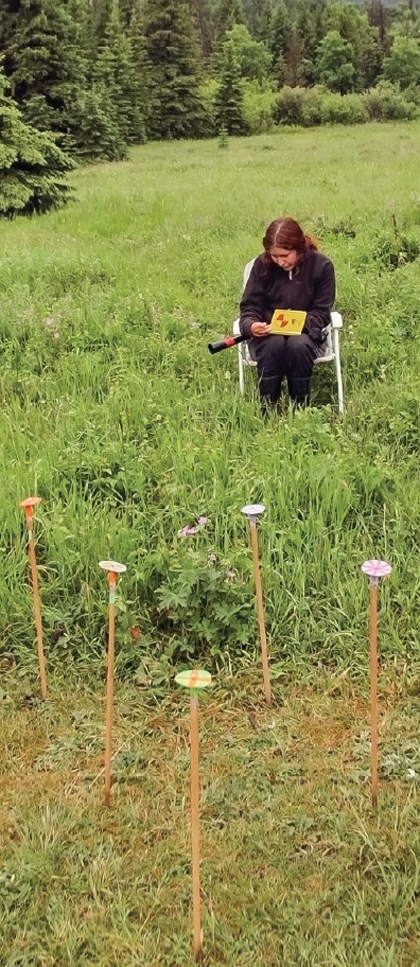
Figure 5. Flowers in an array used by Rachael Marshall (in photo) in one of her timing experiments, showing the proximity of the experimenter to the array. Photo by T. A. Hurly.
The longer we have experimented with these birds, the more we have found evidence for their ability to learn information as is necessary, because we have found they will learn and remember the colours of flowers, we just had to ask them in the appropriate fashion. Two examples will illustrate this. Firstly, in an experiment in which we were primarily interested in the accuracy with which they could remember a location, some birds were trained that yellow flowers were rewarded while others were taught that red flowers were rewarded. This colour-reward association was learned within 2-3 experiences (Hurly & Healy, 1996). Secondly, we trained birds that three of the flowers in an array of ten contained sucrose solution. All of the flowers differed in their colour pattern. Once the birds had learned which were the rewarded flowers we moved the array 2m from the site of the original array so the birds could not use the location of the flowers to determine which were the rewarded flowers. However, it was not until we had also changed the shape of the moved array that we found that the birds had remembered the colours of the flowers rewarded in the first array (Hurly & Healy, 2002; Figure 6). The birds can and will learn and remember colour but our ability to demonstrate that they can and will do so required us to be much more particular about our experimental designs. We would have been both remiss and incorrect if we had concluded that rufous hummingbirds were unable to learn colour cues, a very ubiquitous cognitive ability.
Not only do we have to be particular about our experimental designs, we also have to be careful about our expectations of what these birds may or may not be capable. Expectations of animals’ cognitive abilities tend to come from two sources, some based on knowledge of the animals’ ecology and others from what might loosely be described as being based on their brain size. Rufous hummingbirds weigh around 3g and, although they have a brain that is larger than expected for their body size (Ward, Day, Wilkening, Wylie, Saucier, & Iwaniuk, 2012), that brain is still not very large. Knowledge of the birds’ ecology leads to expectations that these birds might, for example, pay more attention to spatial information than to colour information (but that they would still pay attention to colour in the relevant contexts), while their brain size might lead to expectations of noticeable limits to the capacity for and speed and the accuracy with which the birds learn spatial locations. We are familiar with onetrial learning from the retrieval successes of food-storers and from long-delay taste aversion learning but even in tasks where animals are highly motivated to learn locations such as in rats searching for hidden platforms in the Morris water maze, animals often either take several trials to learn a location or require some time exploring the location in a first visit. Like food-storing birds, rufous hummingbirds, however, learn the three-dimensional location of a reward from a single visit that lasts only a few seconds. They can return to that location even in the absence of the flower (Flores Abreu, Hurly, & Healy, 2012) and they can visit several such ‘empty’ locations. Usefully, hummingbirds can demonstrate their memory for a rewarding location in the absence of the local cues of that reward because they will fly to, and then hover at, that location, much as a rat in a water maze will swim back and forth over the place it has learned to find a hidden platform.
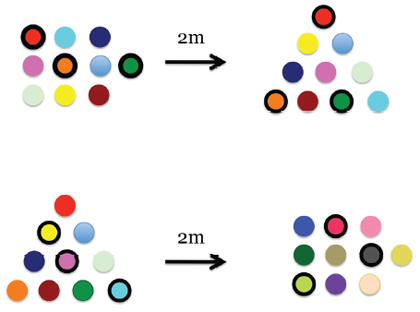
Figure 6. Schematic of the arrays used to determine that the birds did learn and remember colour (redrawn from Healy and Hurly 2002, above – treatment; below – control).
Although we have not measured the accuracy with which the birds can return to a flower that has been removed after a single visit, we have attempted to measure the 3-D accuracy of memories for a familiar location. We trained birds to fly to a rewarded flower (a red 8cm3 cardboard cube) in a large featureless field, then removed the flower and filmed the bird’s flight path into the location of the now-missing flower. The birds flew to within 60cm in the horizontal plane and within 20cm in the vertical plane (Hurly, Franz, & Healy, 2010). They did not appear to beacon to the flower in spite of the ‘flower’ being highly conspicuous, as when we simply moved the flower about 1.5m, the birds flew nearer to the location of the missing flower, and hovered, before flying directly to the moved flower. The birds’ accuracy for a flower’s location seems to depend on the size of the flower. In a second experiment, we trained birds to feed from either a small (8cm3) or a large flower (1000cm3). This time, in the absence of the flower, the birds flew even closer to its previous location than they had in the earlier experiment (the locations were not the same in the two presentations): 20cm in the horizontal and around 5cm in the vertical when the flower was small and around 50cm in the horizontal and about 25cm in the vertical when the flower was large. These data finally allowed us to confirm the precision with which a hummingbird can return to a learned but absent reward described in the many anecdotes of hummingbirds returning to sites of feeders they had fed from during their last migration or breeding season. The appearance of birds at particular windows of houses is a common incentive for people to get feeders out of the cupboard after the winter.
One obvious difference for the birds in our experiments from the birds in these reports, however, was that we deliberately chose to place the experimental flower at least 10m from any obvious landmarks (e.g., bushes, trees; Figures 1 and 5). The data from experiments on various species in the laboratory would suggest that the birds might have learned the flower’s location in one of three ways: they may have learned the visual characteristics of the flower and used it as a beacon, they could have used the landmarks proximal to the flower or, they used a number of distal landmarks. The behaviour of our real-world animals, however, does not readily conform to any of these three possibilities: while they can use the flower as a beacon, as shown by the birds flying to the moved flower once they discover the one in the familiar location is missing, they do not need to beacon to the flower and they do not do so preferentially. Graham, Fauria, & Collett, (2003) suggested that their ants might use large landmarks along a route as beacons while learning that route and that those landmarks might act as a scaffold for learning other landmarks nearby so that the animals could move along the route if the beacons were then removed. While this seems plausible for our hummingbirds it is not at all clear which landmarks along the way would have formed this scaffold. The proximal landmarks were (to our eyes, at least) remarkably uniform: the ground was quite flat, and covered by vegetation that reached perhaps 20cm punctured by multiple ground squirrel burrows. The distal landmarks, on the other hand, were very conspicuous and ranged from trees, typically ringing the open fields used for training and testing, to the mountains rising some 1000m along both sides of the valley and visible from all points in the open fields. However, it was still not clear how such large landmarks would enable the birds to be quite so accurate in their return to the flower location. It is possible that the birds did not use visual cues at all as they may well have used magnetic or sun compass cues instead but there is no reason to believe that such cues would be better at supporting accuracy than are distal visual landmarks. We have no useful information on the use of either of these systems in hummingbirds although there is an abundance of data for their use by other birds so it seems at least plausible that hummingbirds might also use them. We also cannot reject the possibility that the birds did use either proximal or distal visual landmarks simply because we cannot yet determine which they might have used or how they might have used them.
Surprisingly, these data on the memory that hummingbirds have for rewards located in three-dimensional space are among only a handful of data on spatial cognition in 3-D (Grobéty & Schenk, 1992; Holbrook & Burt de Perera, 2009; Holbrook & Burt de Perera, 2011). Although all flying and swimming animals live their lives moving through 3-D space, even terrestrial animals can and will move through the vertical dimension of their world and yet, we know almost nothing about whether the z-dimension is encoded differently from the way in which x and y dimensions are encoded, whether the animals pay attention to the dimensions differently or whether either or both of these are dependent on whether the animal moves through the z-dimension. Rufous hummingbirds might remember flowers bettewr if they differ in their height (Henderson, Hurly, & Healy, 2006b) and they may also remember a 3-D location more accurately than do rats, when both species have learned a location in a 3-D maze (Flores Abreu, 2012). Whether these outcomes are due to greater familiarity with moving through 3-D space or to an ability that has been favoured by natural selection is not yet clear and requires investigation of 3-D spatial performance in more species.
That performance depends on the way in which the question is asked is demonstrated yet again because hummingbirds apparently encode vertical information less accurately than horizontal information when the locations to be discriminated differ only in their vertical component: when flowers were presented on a vertical pole (Figure 7), birds found it difficult to learn which one of five flowers was rewarded but when the flowers were presented along a diagonal pole, the birds were relatively quick to learn which was the rewarded flower (Flores Abreu, Hurly, & Healy, 2013). Here it appears that the addition of a horizontal component to the flower’s location may have facilitated the learning of its vertical location. Additionally, when presented with only two flowers the location of which differed only in the vertical component, hummingbirds appeared to learn which was the rewarded flower relative to the other i.e., whether the flower was the upper or the lower of the two flowers (Henderson et al., 2006b).
Unlike 3-D spatial cognition, a considerable amount of work has been conducted on a variety of species into the ways they learn and use time. Interval timing over short time periods has been well studied in the laboratory while circadian timing has been much investigated in both the laboratory and the field (e.g., Sylvia borin, Biebach, Falk, & Krebs, 1989; pigeons Columba livia, Saksida & Wilkie, 1994; hamsters Mesocricetus auratus, Cain, Chou, & Ralph, 2004; and bees Apis mellifera, Pahl, Zhu, Pix, Tautz, & Zhang, 2007). More recently, investigations into episodiclike memory (often also called what-where-when memory) have also raised questions as to what kind of time constitutes the ‘when’ component of this kind of memory. There are a priori reasons to suppose that rufous hummingbirds might also be capable of using more than one kind of time. For example, it has been suggested that territorial hummingbirds, like the rufous, use defence by exploitation as a means to exclude nectarivorous intruders and that this is effected by the territorial holder feeding on, and thereby emptying, the flowers at the edge of his territory early in the day (Paton & Carpenter, 1984). Traplining, whereby an animal moves around a circuit of resources (such as flowers) in a predictable pattern and time period, may also be used by foraging hummingbirds that are using their floral resources effectively (Feinsinger & Colwell, 1978). Finally, for hummingbirds foraging on flowers that refill their nectar supplies (perhaps within a few hours), being able to remember which flowers they visited and when, would enable more effective foraging. The biology of these birds, then, suggests that they might be capable of circadian timing, sequence learning and/ or interval timing.
Given the possible daily requirement for remembering when flowers had been emptied, we began investigating the hummingbirds’ ability to learn time intervals by presenting a bird with an array of eight, individually distinctive flowers, four of which were refilled 10 minutes after the bird had emptied them and four were refilled 20 minutes after being emptied. Each flower had contained the same amount and concentration of sucrose so the time to refill was not an indicator of a flower’s contents and the amount in a flower was such that the bird would typically visit 3-5 flowers per visit to the array. However, he could visit all the flowers or only one, the number of flowers and which flowers to visit were chosen by the bird. The array was presented in the morning and the territory owner was allowed to visit at will throughout the day. The flowers were removed overnight and replaced in the same place the following day. As we did not know how long it would take for a bird to learn the refill rates (if at all), we presented each of three territorial birds with an eight-flower array for 11 days. It turned out that the birds had, in fact, learned the refill rates quite well by the end of the first day (our expectations of their performance vastly underestimated their abilities) but the key finding was that all three birds returned to the 10-minute refilling flowers at around 10 minutes and to the 20-minute refilling flowers at around 20 minutes (Henderson et al., 2006a). Although these time periods are much shorter than typical refilling times for real flowers and birds may defend up to a couple of thousand flowers in their territories, we considered that the Henderson et al. (2006a) data at least showed ‘proof of principle’ and that these birds could learn refill rates, for multiple flowers and relatively quickly. Furthermore, not only were the duration of the refill rates longer and the number of flowers that the birds could track more than has been tested in the laboratory, all of the birds were living a very active life throughout their foraging on the array, defending their territory from intruding males and displaying to females. One suspects that if these birds were tested in the more controlled environment of a laboratory, their abilities would seem even more impressive. Rather than testing them in the laboratory, we then looked to see whether, if the colour of the flower signalled the refill rate, the birds would use that colour and learn the refill rate of the flower more quickly than if there was no colour-refill association. This was one of the instances in which we based our expectation of what the birds would learn on the information we would be likely to use ourselves: if the 10-minute refilling flowers were blue and the 20-minute refilling flowers were pink, for example, it would seem that the colour might reduce the time taken to learn the refill schedule. However, it did not affect the speed at which the birds learned which flowers refilled after 10 minutes and which refilled after 20 minutes (Marshall, Hurly, & Healy, 2012; Figure 5). Indeed, there is some preliminary evidence that colour-refill associations may also not affect the speed with which people learn the duration before which flowers refill (Marshall, 2012). In a second experiment reported by Marshall et al., (2012), birds also did not learn which flowers held 20% sucrose solution and which held 30% sucrose sooner when the flower colour signalled the sucrose concentration. Of course, our earlier data whereby birds did not appear to learn the colour of a flower when its location did not change should have alerted us to the probability that colour would also not be salient to the birds in the Marshall et al. (2012) experiment, or at least, not as salient as is space. It appears that space overshadows colour information in a range of contexts. One way to test this would be to move the flowers after each visit. In such a manipulation, space would no longer be a reliable cue and colour would be.
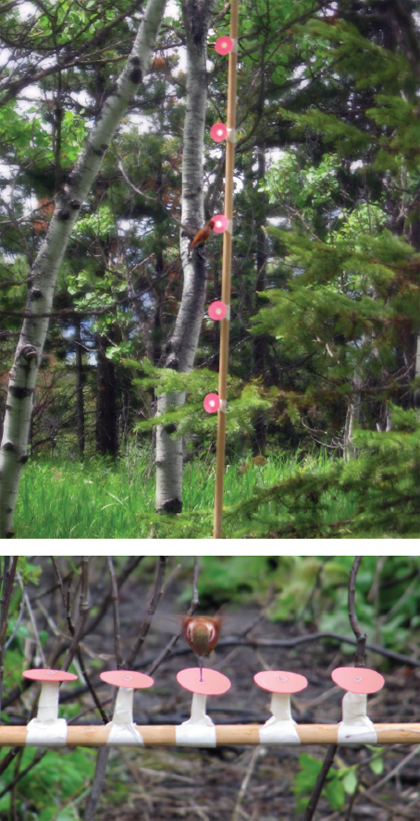
Figure 7. Photographs showing the flower arrays used by Ileana Nuri Flores Abreu to examine the use by the hummingbirds of horizontal and vertical information. Photos by I. N. Flores Abreu.
Having found that the hummingbirds could learn refill rates, and in experiments on risk sensitivity and context-dependent choice, that they readily learn what (volume and concentration of sucrose) is held in flowers or wells of different colours, it was clear that these birds could learn and remember each of the three (what-where-when) components of episodic-like memory (Hurly & Oseen, 1999; Bateson, Healy, & Hurly, 2002; 2003; Morgan, Hurly, Bateson, Asher, & Healy, 2012). They can also remember pairs of these components: they can remember when and where (Henderson et al., 2006a) and they can remember what and where (e.g., Healy & Hurly 1995; 1998) although we have not explicitly looked for whether they can remember what and when together. The question then was whether they could remember all three components together. Although there is now considerable evidence that a range of animals have episodic-like memory, including rats (Babb & Crystal, 2005), magpies (Zinkivskay et al., 2009), chickadees (Feeney et al., 2009), and meadow voles (Ferkin et al., 2008), issues over designing an appropriate experiment continue to plague this field. The most systematic difficulty concerns the ‘when’ component, with differing groups defining this in different ways (e.g., including a place in a sequence: Ergorul & Eichenbaum, 2004; a time of day: Zhou & Crystal, 2009; and using “which” instead of “when”: Eacott, Easton, Zinkivskay, 2005; Eacott & Norman, 2004). Common to all of these approaches, however, is that the test would allow animals to demonstrate their ability to remember what, where and when in combination. We took a slightly different approach again, which was to design an experiment in which we could explicitly examine all of the components of whatwhere and when memory. We did this by presenting birds with a pair of four-flowers arrays in which the single flower that contained reward differed for the different times of day at which the arrays were presented. The distinctive colours of the four flowers in each array (e.g., blue, yellow, purple, and red; Figure 8) occurred in the same relative positions for both arrays. The arrays were presented in the morning and a territorial male was allowed to search around the flowers to find the rewarded flower. He was then allowed to return to the arrays to feed from the rewarded flower for a further five visits before the arrays were removed. The arrays were presented again in the afternoon and the bird had again to find the rewarded flower. In this latter array presentation, the rewarded flower was in the other array and of one of the other colours. After five subsequent visits to the rewarded flower, the arrays were removed. They were presented to the bird over the next few days at approximately the same times each day. Over the course of these presentations we found that birds visited the eight different flowers differently: there was a single ‘correct’ flower, which was in the correct array at the correct time and of the correct colour, one flower that was in the correct array and of the correct colour but at the wrong time, one flower that was at the correct time and of the correct colour but the wrong array, three flowers that were in the correct array at the correct time, and two flowers that were wrong array, at the wrong time and of the wrong colour. The most common flower the birds chose was the rewarded flower and they went least frequently to the flowers that were completely wrong (Marshall, 2012). Of the other kinds of flowers, relative to chance, the birds went most often to the flower that was rewarded at the other time of day, which is consistent with the data from episodic-like memory tests where it is the when component that is the most difficult for the animal to get right. Furthermore, the birds most readily corrected what errors as they typically flew from a what error flower directly to the correct flower. These outcomes are consistent with what we have seen in other experiments with the birds: they have good spatial memory and they seem to remember colour only when space is not relevant. Although this experiment was not an episodic-like memory experiment as the birds visited the arrays six times at each time period and the trials were not trial-unique, this kind of experimental design might enable a comparison across species in their episodic-like memories of how well they can remember each of the three components. For example, do other animals remember the where better than the what and the when as do hummingbirds? Might a species’ ecology enable us to predict the pattern of variation in the structure of episodic-like memories (if there is one)? It might also help to determine which species serves as the most useful model of human episodic memories. The data from this experiment also suggest that, in addition to being capable of learning intervals, the birds can learn circadian time.
One-trial learning of 3-D locations, accurate spatial memory and timing capacity: these animals have cognitive capabilities that appear to match their ecological requirements, although we have not actually tested the extent of these abilities (e.g., whether the birds can remember most of the flowers in their territories, rather than the handful on which we have tested them). For us, this raises multiple questions, such as how specific are these abilities? Is it the case, for example, that other animals are also capable of this kind of timing capacity or has natural selection favoured this particularly in the hummingbirds and only in other species that have faced similar cognitive challenges in their foraging strategy (or in some other part of their lifestyle)? This question requires comparative data, of the kind gathered to address similar questions asked of the capacities of food storers relative to nonstorers, of sticklebacks living in different environments, and a handful of other species (Girvan & Braithwaite, 1998; McGregor & Healy, 1999; Odling-Smee, Boughman, & Braithwaite, 2008).
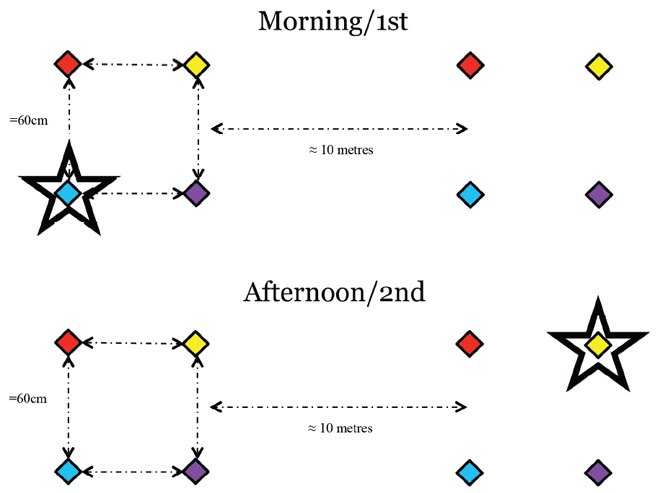
Figure 8. Schematic of the what-where-when experiment in which time of day was the ‘when’.
Accurate spatial memory and timing capabilities allow rufous hummingbirds to make appropriate decisions about which flowers to visit and when and we have used their ecology to make predictions about their cognitive capabilities. We have also used data from the literature on human decision-making to ask questions about whether or not these birds make so-called irrational decisions. A rational decision is one in which the animal/human faced with an array of options is expected to choose the option that obtains the highest utility. For animals, the utility of a foraging option is considered to be the one that provides the highest energetic return. For humans, utility might be measured in terms of energy, finance, or some other useful currency and humans were also always assumed to make rational choices. However, there is now a wealth of data to show that humans do not always make rational decisions and increasing evidence to show that animals, including rufous hummingbirds, also do not make such decisions (Bateson et al., 2002; 2003; Hurly & Oseen, 1999; Latty & Beekman, 2011; Morgan et al., 2012; Sasaki & Pratt, 2011; Scarpi, 2011, Waite, 2001). One of the experimental paradigms used to show that rufous hummingbirds are irrational consists of offering birds a choice between two favourable options (a binary choice set; Figure 2) and a choice among three options, the two favourable options from the binary choice set plus a third poorer option. If the birds were choosing rationally, the inclusion of the poorer option should not affect the relative preference the birds have for the two favourable options, but it does (Bateson et al., 2002; 2003; Morgan et al., 2012). One suggested mechanism for this change in preference is that sampling of the poorer option forces birds to take relatively more of the better of the two favourable options to regain the lost energy. However, the hummingbirds do not always respond to the poorer option by increasing their preference for the option with the highest energy return (Morgan et al., 2012). Another possibility is that the birds assess the options available relative to each other rather than with respect to their absolute values. This might mean then, that the inclusion of a poor option in the choice set might make the better of two favourable options seem much better than when just the two favourable options are presented and so a bird should increase its relative preference for that better option. It might also mean that the inclusion of very poor option to the choice set would lead the bird to perceiving the two favourable options as more similar to each other, which would result in a bird choosing the two favourable options more similarly than it had when presented with the binary choice. Just this experiment is currently underway.
Why hummingbirds might make irrational decisions over foraging options is not yet clear. There are at least two possibilities that have been raised to explain irrational human decision making: 1) the birds are perceptually or cognitively constrained and are simply unable to measure each of the options sufficiently accurately to make the appropriate (rational) choice; 2) the birds are capable of measuring the available options but trade off making the perfect decision with the time taken to make the measurements as the costs of making the perfect decision at every foraging choice (for hummingbirds around every 10 minutes through the day) well outweigh the benefits of that decision. This latter seems intuitively more likely as no one foraging decision for these birds will be worth a bird spending a lot of time assessing the options. However, whether the frequent foraging for small meals means that these birds are more likely to make irrational decisions than are animals making more substantive decisions (in fitness terms), such as mate choice or offspring sex ratio is not yet clear. This is also a question that would be best addressed by a comparative approach, both within and among species. For example, rufous hummingbirds choosing mates may not trade off time with assessment accuracy because this is a decision that has medium to long-term fitness effects. Similarly, animals that feed on few but large meals as do many top predators may also be prepared to take longer to choose among foraging options in order to ensure they choose the option with the highest energetic return.
More comparative data would help to determine the role that cognition plays in the lives of animals and that is played by natural selection in producing variation in cognitive abilities. We are beginning to determine the cognitive capabilities of rufous hummingbirds when those animals are living a fast and furious life in the midst of our experiments. We consider that this work brings us considerably closer to understanding the use to which these animals could put their cognitive abilities than if we had conducted this work in the laboratory. In the real world, the benefits to cognitive abilities might range from the short term such as finding food for a single meal to the medium term such as managing to attract a good mate (Keagy, Savard, and Borgia, 2009), with the ultimate goal of the production of more and/or better offspring. Demonstration of these benefits requires not only the measurement of that ability, it also requires measurement of how variability in that ability maps onto a tangible benefit. However, although most assume that being ‘smarter’ must be beneficial, the data are remarkably sparse. Indeed, to our knowledge, perhaps the first data that might begin to confirm the smarter is better assumption have only just been published (Cole, Cram, & Quinn, 2012). Cole et al. (2012) showed that great tits (Parus major) brought into the laboratory for testing, that learned how to access food from a manmade dispenser, when returned to the wild, went on to lay more eggs over the following four years. These birds also managed to spend less time feeding their offspring. To show these effects Cole et al. (2012) tested, and then tracked in the field, over 400 individuals, a feat that many will find difficult to emulate. However, while laying eggs would suggest actual fitness benefits to greater problem-solving abilities, the solver great tits were also more likely to desert their nests than were those tits that did not solve the food access problem. In the medium term, then, problem-solving great tits did not produce more grand-offspring than did the nonsolvers.
We (collectively) have a way to go before we have good evidence for fitness benefits of cognitive abilities. However, we hope that our focus on our work on rufous hummingbirds shows that one can usefully address questions about cognitive abilities in wild, free-living animals. Furthermore, these data provide tentative evidence for natural selection acting on cognitive abilities: like food-storing songbirds and unlike non-food storing songbirds (e.g., Brodbeck, 1994), rufous hummingbirds place greater emphasis on spatial cues over colour cues, a hierarchy of cue preference that is correlated with their particular ecological demands. However, it remains to be seen whether other animals, living very different lives, will pay attention to time as do the hummingbirds. Finally, if natural selection has shaped cognitive abilities as it appears, then the next challenge will be for us to determine what cognitive capabilities hummingbirds lack.
References
Altshuler, D.L. (2003). Flower color, hummingbird pollination, and habitat irradiance in four Neotropical forests. Biotropica, 35 , 344-355. doi.org/10.1111/j.1744-7429.2003.tb00588.x doi.org/10.1646/02113
Auersperg, A. M. I., Huber, L., & Gajdon, G. K. (2011). Navigating a tool end in a specific direction: stick-tool use in kea (Nestor notabilis). Biology Letters, 7, 825-828. doi.org/10.1098/rsbl.2011.0388 PMid:21636657 PMCid:3210666
Babb, S. J. & Crystal, J. D. 2005. Discrimination of what, when, and where: Implications for episodic-like memory in rats. Learning and Motivation, 36, 177-189. doi.org/10.1016/j.lmot.2005.02.009
Balda, R.P., Pepperberg, I.M., & Kamil, A.C. 1998. Animal cognition in nature: The convergence of psychology and biology in laboratory and field. Academic Press. PMCid:1170538
Bateson, M., Healy, S.D. & Hurly, T.A. 2002. Irrational choices in hummingbird foraging behaviour. Animal Behaviour, 63, 587-596. doi.org/10.1006/anbe.2001.1925
Bateson, M., Healy, S.D. & Hurly, T.A. 2003. Context-dependent foraging decisions in rufous hummingbirds. Proceedings of the Royal Society London B 270, 1271-1276. doi.org/10.1098/rspb.2003.2365 PMid:12816640 PMCid:1691372
Biebach, H., Falk, H. & Krebs, J. R. (1991). The effect of constant light and phase shifts on a learned time-place association in Garden Warblers (Sylvia borin): Hourglass or circadian clock? Journal of Biological Rhythms, 6, 353- 365. doi.org/10.1177/074873049100600406 PMid:1773101
Biegler, R, McGregor, A, Krebs, J.R. & Healy, S.D. 2001. A larger hippocampus is associated with longer lasting spatial memory. Proceedings of the National Academy of Sciences USA, 98, 6941-6944. doi.org/10.1073/pnas.121034798 PMid:11391008 PMCid:34457
Boogert, N.J., Fawcett, T.W. & Lefebvre, L. 2011 . Mate choice for cognitive traits: a review of the evidence in nonhuman vertebrates. Behavioral Ecology, 22, 447-459. doi.org/10.1093/beheco/arq173
Briscoe, A.D. & Chittka, L. 2001. The evolution of color vision in insects. Annual Review of Entomology, 46, 471-510. doi.org/10.1146/annurev.ento.46.1.471 PMid:11112177
Brodbeck, D. R. (1994). Memory for spatial and local cues – a comparison of a storing and a nonstoring species. Animal Learning & Behavior, 22, 119-133. doi.org/10.3758/BF03199912
Cain, S. W., Chou, T. & Ralph, M. R. 2004. Circadian modulation of performance on an aversion-based place learning task in hamsters. Behavioural Brain Research, 150, 201-205. doi.org/10.1016/j.bbr.2003.07.001 PMid:15033293
Clark, C. J. 2009. Courtship dives of Anna’s hummingbird offer insights into flight performance limits. Proceedings of the Royal Society London B, 276, 3047-3052. doi.org/10.1098/rspb.2009.0508 PMid:19515669 PMCid:2817121
Clayton, N. S. & Dickinson, A. (1999). Memory for the content of caches by scrub jays (Aphelocoma coerulescens). Journal of Experimental Psychology: Animal Behavior Processes, 25, 82-91. doi.org/10.1037/0097-7403.25.1.82 PMid:9987859
Cole, E.F., Cram, D.L. & Quinn, J.L. 2011. Individual variation in spontaneous problem-solving performance among great tits. Animal Behaviour, 81, 491-498. doi.org/10.1016/j.anbehav.2010.11.025
Dally, J. M., Emery, N. J. & Clayton, N. S. 2010. Avian Theory of Mind and counter espionage by food-caching western scrub-jays (Aphelocoma californica). European Journal of Developmental Psychology, 7, 17-37. doi.org/10.1080/17405620802571711
Eacott, M. J., Easton, A. & Zinkivskay, A. 2005. Recollection in an episodic-like memory task in the rat. Learning & Memory, 12, 221-223. doi.org/10.1101/lm.92505 PMid:15897259
Eacott, M. J. & Norman, G. 2004. Integrated memory for object, place, and context in rats: A possible model of episodic-like memory? Journal of Neuroscience, 24, 1948-1953. doi.org/10.1523/JNEUROSCI.2975-03.200 PMid:14985436
Ergorul, C. & Eichenbaum, H. 2004. The hippocampus and memory for “What,” “Where,”” and “When”. Learning & Memory, 11, 397-405. doi.org/10.1101/lm.73304 PMid:15254219 PMCid:498318
Feeney, M., Roberts, W. & Sherry, D. 2009. Memory for what, where, and when in the black-capped chickadee (Poecile atricapillus). Animal Cognition, 12, 767-777. doi.org/10.1007/s10071-009-0236-x PMid:19466468
Feinsinger, P. & Colwell, R. K. (1978). Community organization among neotropical nectar-feeding birds. American Zoologist, 18, 779-795.
Ferkin, M. H., Combs, A., Delbarco-Trillo, J., Pierce, A. A. & Franklin, S. 2008. Meadow voles, Microtus pennsylvanicus, have the capacity to recall the “what”, “where”, and “when” of a single past event. Animal Cognition, 11, 147-159. doi.org/10.1007/s10071-007-0101-8 PMid:17653778
Flores-Abreu, I. N. 2012. Spatial cognition in three dimensions. Unpublished PhD thesis, University of St Andrews, UK. Flores-Abreu, I. N., Hurly, T.A. & Healy, S.D. 2012. Onetrial spatial learning: wild hummingbirds relocate a rewarding location after a single visit. Animal Cognition, 15, 631-637. doi.org/10.1007/s10071-012-0491-0 PMid:22526688
Flores-Abreu, I. N., Hurly, T.A. & Healy, S.D. 2013. Three-dimensional spatial learning in hummingbirds. Animal Behaviour. doi.org/10.1016/j.anbehav.2012.12.019
Freas, C.A., LaDage, L.D., Roth, T.C. & Pravosudov, V.V. 2012. Elevation-related differences in memory and the hippocampus in mountain chickadees, Poecile gambeli. Animal Behaviour, 84, 121-127. doi.org/10.1016/j.anbehav.2012.04.018
Girvan, J. R. & Braithwaite, V. A. 1998. Population differences in spatial learning in three-spined sticklebacks. Proceedings of the Royal Society London B, 265, 913-918. doi.org/10.1098/rspb.1998.0378 PMCid:1689060
Graham, P., Fauria, K. & Collett, T.S. 2003. The influence of beacon-aiming on the routes of wood ants. Journal of Experimental Biology, 206, 535-541. doi.org/10.1242/jeb.00115 PMid:12502774
Grobéty, M.-C. & F. Schenk. 1992. Spatial learning in a three-dimensional maze. Animal Behaviour, 43, 1011-1020. doi.org/10.1016/S0003-3472(06)80014-X
Hampton, R. R. & Shettleworth, S. J. 1996. Hippocampus and memory in a food-storing and in a nonstoring bird species. Behavioral Neuroscience, 110, 946-964. doi.org/10.1037/0735-7044.110.5.946 PMid:8918998
Healy, S.D. & Hurly, T.A. 2004. Spatial learning and memory in birds. Brain Behavior and Evolution, 63, 211-220. doi.org/10.1159/000076782 PMid:15084814
Healy, S.D. & Hurly, T.A. 2003. Cognitive ecology: foraging in hummingbirds as a model system. Advances in the Study of Behavior, 32, 325-359. doi.org/10.1016/S0065-3454(03)01007-6
Healy, S.D. & Hurly T.A. 2001. Foraging and spatial learning in hummingbirds. In: Pollination Biology. (Ed. by L. Chittka & J. Thomson). Pp. 127-147. Cambridge University Press. PMid:11446794
Healy, S.D. & Hurly, T.A. 1998. Rufous hummingbirds’ (Selasphorus rufus) memory for flowers: patterns or actual spatial locations? Journal of Experimental Psychology: Animal Behavior Processes, 24, 1-9. doi.org/10.1037/0097-7403.24.4.396
Healy, S.D. & Hurly, T.A. 1995. Spatial memory in rufous hummingbirds (Selasphorus rufus): a field test. Animal Learning and Behavior, 23, 63-68. doi.org/10.3758/BF03198016
Henderson, J., Hurly, T.A., Bateson, M. & Healy, S.D. 2006a. Timing in free-living rufous hummingbirds Selasphorus rufus. Current Biology 16, 512-515. doi.org/10.1016/j.cub.2006.01.054 PMid:16527747
Henderson, J., Hurly, T.A. & Healy, S.D. 2006b. Spatial relational learning in rufous hummingbirds (Selasphorus rufus). Animal Cognition 9, 201-205. doi.org/10.1007/s10071-006-0021-z PMid:16767469
Henderson, J., Hurly, T.A. & Healy, S.D. 2001. Rufous hummingbirds’ memory for flower features. Animal Behaviour, 61, 98-106. doi.org/10.1006/anbe.2000.1670
Holbrook, R. & Burt de Perera, T. 2011. Three-dimensional spatial cognition: information in the vertical dimension overrides information from the horizontal. Animal Cognition, 14, 613-619. doi.org/10.1007/s10071-011-0393-6 PMid:21452048
Holbrook, R. I. & Burt de Perera, T. 2009. Separate encoding of vertical and horizontal components of space during orientation in fish. Animal Behaviour 78, 241-245 doi.org/10.1016/j.anbehav.2009.03.021
Hurly, A. T. 1996. Spatial memory in rufous hummingbirds: memory for rewarded and non-rewarded sites. Animal Behaviour, 51, 177-183. doi.org/10.1006/anbe.1996.0015
Hurly, T.A. & Healy, S.D. 2002. Cue learning by rufous hummingbirds Selasphorus rufus. Journal of Experimental Psychology:Animal Behavior Processes, 28, 209-223. doi.org/10.1037/0097-7403.28.2.209 PMid:11987877
Hurly, T.A. & Healy, S.D. 1996. Memory for flowers in rufous hummingbirds: Location or local visual cues? Animal Behaviour, 51, 1149-1157. doi.org/10.1006/anbe.1996.0116
Hurly, T. A. & Oseen, M. D. 1999. Context-dependent, risk-sensitive foraging preferences in wild rufous hummingbirds. Animal Behaviour, 58, 59-66. doi.org/10.1006/anbe.1999.1130 PMid:10413541
Hurly, T.A., Franz, S. & Healy, S.D. 2010. Do rufus hummingbirds (Selasphorus rufus) use visual beacons? Animal Cognition, 13, 377-383. doi.org/10.1007/s10071-009-0280-6 PMid:19768647
Hurly, T.A., Scott, R.D. & Healy, S.D. 2001. The function of displays in male rufous hummingbirds. The Condor, 103, 647-651. doi.org/10.1650/0010-5422(2001)103[0647:TFODOM]2.0.CO;2
Keagy, J., Savard, J.-F. & Borgia, G. 2012 . Cognitive ability and the evolution of multiple behavioral display traits. Behavioral Ecology, 23, 448-456. doi.org/10.1093/beheco/arr211
Keagy, J., Savard, J. -F., & Borgia, G. (2009). Male satin bowerbird problem-solving ability predicts mating success. Animal Behaviour, 78, 809-817. doi.org/10.1016/j.anbehav.2009.07.011
Latty, T. & Beekman, M. 2011. Irrational decisionmaking in an amoeboid organism: transitivity and context-dependent preferences. Proceedings of the Royal Society London B, 278, 307-312. doi.org/10.1098/rspb.2010.1045 PMid:20702460 PMCid:3013386
Marshall, R.E.S. 2012. Timing and episodic-like memory in the rufous hummingbird. Unpublished PhD thesis, University of St Andrews, UK.
Marshall, R.E.S., Hurly, T.A. & Healy, S.D. 2012. Do a flower’s features help hummingbirds to learn its contents and refill rate? Animal Behaviour, 83, 1163-1169. doi.org/10.1016/j.anbehav.2012.02.003
McGregor, A. & Healy, S.D. 1999. Spatial accuracy in food-storing and nonstoring birds. Animal Behaviour, 58, 727-734. doi.org/10.1006/anbe.1999.1190 PMid:10512645
Miller, R.S. & Miller, R.E. 1971. Feeding activity and color preference of ruby-throated hummingbirds. Condor, 73, 309-313. doi.org/10.2307/1365757
Miller, R.S., Tamm, S., Sutherland, G.D., & Gass C.L. (1985) Cues for orientation in hummingbird foraging: color and position. Canadian Journal of Zoology 63,18–21. doi.org/10.1139/z85-004
Morgan, K.V., Hurly, T.A., Bateson, M., Asher, L., & Healy, S.D. 2012. Context-dependent decisions among options varying in a single dimension. Behavioural Processes, 89, 115-120. doi.org/10.1016/j.beproc.2011.08.017 PMid:21945144
Muth, F. & Healy, S.D. 2011. The role of adult experience in nest building in the zebra finch, Taeniopygia guttata. Animal Behaviour, 82, 185-189. doi.org/10.1016/j.anbehav.2011.04.021
Odling-Smee, L.C., Boughman, J.W. & Braithwaite, V.A. 2008. Sympatric species of threespine stickleback differ in their performance in a spatial learning task. Behavioral Ecology and Sociobiology, 62, 1935-1945. doi.org/10.1007/s00265-008-0625-1
Pahl, M., Zhu, H., Pix, W., Tautz, J. & Zhang, S. W. 2007. Circadian timed episodic-like memory – a bee knows what to do when, and also where. Journal of Experimental Biology, 210, 3559-3567. doi.org/10.1242/jeb.005488 PMid:17921157
Paton, D .C. & Carpenter, F. L. (1984). Peripheral foraging by territorial rufous hummingbirds – defense by exploitation. Ecology, 65, 1808-1819. doi.org/10.2307/1937777
Raven, P.H. 1972. Why are bird-visited flowers predominantly red. Evolution, 26, 674-674. doi.org/10.2307/2407064
Saksida, L. & Wilkie, D. 1994. Time-of-day discrimination by pigeons, Columba livia. Learning & Behavior, 22, 143-154. doi.org/10.3758/BF03199914
Sasaki, T. & Pratt, S. C. 2011. Emergence of group rationality from irrational individuals. Behavioral Ecology, 22, 276-281. doi.org/10.1093/beheco/arq198
Scarpi, D. 2011. The impact of phantom decoys on choices in cats. Animal Cognition, 14, 127-136. doi.org/10.1007/s10071-010-0350-9 PMid:20838836
Schmidt, J., Scheid, C., Kotrschal, K, Bugnyar, T. & Schloegl, C. 2011. Gaze direction – A cue for hidden food in rooks ( Corvus frugilegus)? Behavioural Processes, 88, 88-93. doi.org/10.1016/j.beproc.2011.08.002 PMid:21855614 PMCid:3185283
Seed, A. & Byrne, R. 2010. Animal Tool-Use. Current Biology, 20, R1032-R1039. doi.org/10.1016/j.cub.2010.09.042 PMid:21145022
Sherry, D. F. & Vaccarino, A. L. 1989. Hippocampus and memory for food caches in black-capped chickadees. Behavioral Neuroscience, 103, 308-318. doi.org/10.1037/0735-7044.103.2.308
Taylor, A.H., Elliffe, D., Hunt, G.R. & Gray, R.D. 2010. Complex cognition and behavioural innovation in New Caledonian crows. Proceedings of the Royal Society London B, 277, 2637-2643. doi.org/10.1098/rspb.2010.0285 PMid:20410040 PMCid:2982037
Teschke, I. & Tebbich, S. 2011. Physical cognition and tool-use: performance of Darwin’s finches in the two trap tube task. Animal Cognition, 14, 555-563. doi.org/10.1007/s10071-011-0390-9 PMid:21360118
van Casteren, A., Sellers, W. I., Thorpe, S. K. S., Coward, S., Crompton, R.H., Myatt, J.P. & Ennos, A.R. 2012. Nest-building orangutans demonstrate engineering know-how to produce safe, comfortable beds. Proceedings of the National Academy of Sciences USA, 109, 6873-6877. doi.org/10.1073/pnas.1200902109 PMid:22509022 PMCid:3344992
Waite, T. A. 2001. Background context and decision making in hoarding gray gays. B ehavioral Ecology, 12, 318-324. doi.org/10.1093/beheco/12.3.318
Walsh, P., Hansell, M. & Healy, S.D. 2010. Repeatability of nest morphology in African weaverbirds. Biology Letters, 6, 149-151. doi.org/10.1098/rsbl.2009.0664 PMid:19846449 PMCid:2865054
Walsh, P.T., Hansell, M. Borello, W. & Healy, S.D. 2011. Individuality in nest building: do Southern Masked weaver (Ploceus velatus) males vary in their nest-building behavior? Behavioural Processes, 88, 1-6. doi.org/10.1016/j.beproc.2011.06.011 PMid:21736928
Ward, B.J., Day, L.B., Wilkening, S.R., Wylie, D.R., Saucier, D.M., & Iwaniuk, A.N. 2012. Hummingbirds have a greatly enlarged hippocampal formation. Biology Letters, 8, 657-659. doi.org/10.1098/rsbl.2011.1180 PMid:22357941
Weir, A.A.S., Chappell, J. & Kacelnik, A. 2002. Shaping of hooks in New Caledonian crows. Science 297, 981-981. doi.org/10.1126/science.1073433 PMid:12169726
Zhou, W. & Crystal, J. D. 2009. Evidence for remembering when events occurred in a rodent model of episodic memory. Proceedings of the National Academy of Sciences, 106, 9525-9529. doi.org/10.1073/pnas.0904360106 PMid:19458264 PMCid:2695044
Zinkivskay, A., Nazir, F. & Smulders, T. V. 2009. What-Where-When memory in magpies (Pica pica). Animal Cognition, 12, 119-125. doi.org/10.1007/s10071-008-0176-x PMid:18670793